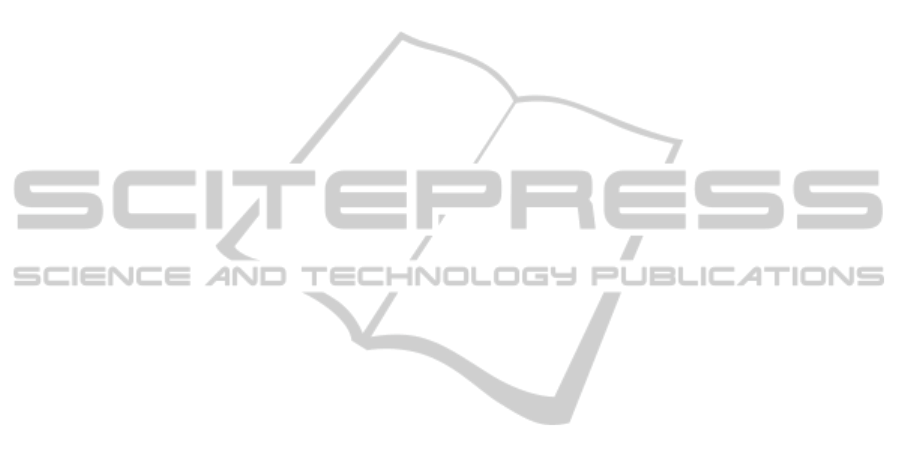
scenario.
The path arrival time for the 1
st
path is almost the
same for all the confined spaces. For Floor G and
Floor 1 the other paths arrive at a larger time because
of NLOS in Floor G and open space in Floor 1.
The channel statistics show that for Floor LA and
Floor G we have a large excess delay and rms delay
spread, resulting in a smaller coherence bandwidth.
Whereas for the Floor 1 and B3 we have a smaller
excess delay and higher coherence bandwidth.
Diffraction loss has also been computed theoreti-
cally for Floor 1 using the Knife edge geometry and
practically taking the power difference. There is a dif-
ference of 6 dB in the results which is due to the ap-
proximation in the theoretical model.
ACKNOWLEDGEMENTS
This work was carried out under SABIC Fast track
project SB-00910. The authors would like to thank
King Fahd University of Petroleum and Minerals for
this research opportunity.
REFERENCES
A. Chandra, A. Kumar, P. (1999). Comparative study of
path losses from propagation measurements at 450
mhz, 900 mhz, 1.35 ghz and 1.89 ghz in the corri-
dors of a multifloor laboratory-cum-office building. In
IEEE Vehicular Technology Conference (VTC 1999-
Fall), Amsterdam, the Netherlands.
Abdellah Chehri, Paul Fortier, P. M. T. Characterization of
the ultra-wideband channel in confined environments
with diffracting rough surfaces. In Wireless Pers Com-
mun DOI 10.1007/s11277-010-0097-2.
Asrar Sheikh, Muhammad Imran Akram, M. K. (2010).
Wireless channel characterization in an indoor en-
vironment. In The 13th International Symposium
on Wireless Personal Multimedia Communications
(WPMC 2010).
E., D. L. O. (Jan. 1952). Propagation studies at microwave
frequencies by means of very short pulses. In BSTJ,
pp. 91-103.
Hawbaker, D. and T.S.Rappaport (1990). Indoor wideband
radiowave propagation measurements at 1.3 ghz and
4.0 ghz. In Electronics Letters,Vol.26,No. 1.
H.Hashemi (July 1993). The indoor radio propagation
channe1. In Proceedings of the IEEE,Vol.81 No.7 pp.
943- 968.
J. Francois, M. L. (1990). Measurement and modeling of
propagation loses in a building at 900 mhz. In IEEE
Transaction on vehicular technology, Vol.39,No. 2.
Poon, A. S. Y. and Ho, M. (2003). Indoor multiple-antenna
channel characterization from 2 to 8 ghz. In ICommu-
nications and Interconnect Lab, Intel Corporation.
SALOUS, S. H. (1999). Wideband mobile radio channel
characterization. In Annals of Telecommunications,
Volume 54, Numbers 1-2, 103-11.
Seidel, S. and Rappaport, T. (1992). 914 mhz path loss pre-
diction models for wireless communications in multi-
floored buildings. In IEEE Trans. Antennas Propaga-
tion. Vol.40, No.2, pp.207-217.
Simon Chiu, J. C. and Michelson, D. G. (March 2010).
Characterization of uwb channel impulse responses
within the passenger cabin of a boeing 737-200 air-
craft. In IEEE Transactions on Antennas and Propa-
gation page:935 vol:58 issue:3.
T.S.Rappaport (2002). Wireless Communications: Princi-
ples and Practice. Prentice Hall, 2nd edition.
Turin G. L., Clapp E. D., J. T. L. F. S. B. L. D. (1972). A
statistical model of urban multipath propagation. In
IEEE Trans. on VT, 21, pp. 1-9.
U. Dersch, J. T. and Zollinger, E. (1994). Multiple reflec-
tions of radio waves in a corridor. In IEEE Trans.
on Antenna and Propagation,Vol. 42, No.9, pp. 157
1- 1574.
X. Zhao, J. Kivinen, P. V. K. S. (April 2002). Propagation
characteristics for wideband outdoor mobile commu-
nications at 5.3 ghz. In IEEE Journal on selected ar-
eas in communications, vol. 20, NO. 3.
Young W. R., L. L. Y. (March 1950). Echoes in transmission
at 450 megacycles from land-to-car radio units. In
Proc. IRE, 38, pp. 255-258.
WINSYS 2011 - International Conference on Wireless Information Networks and Systems
108