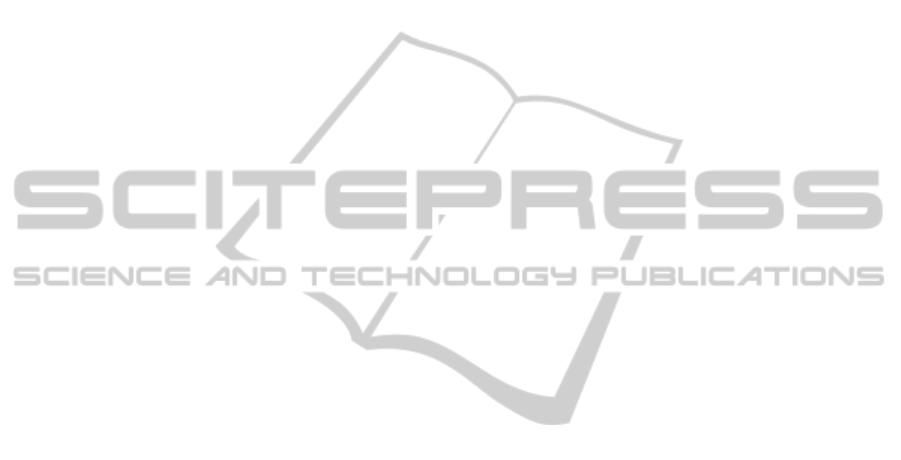
UWB snapshots at the output of the BPF used in each
branch of the electrical receiver of the WDM Ph-ADC
system. Fig. 9 and 10 confirm that time stretching
of the signals at the different branches (and that have
been transmitted in different optical wavelengths) of
the electrical receiver is reached when the WDM ar-
chitecture is employed. Notice that the OFDM-UWB
radio signals centre frequency initially captured by
the sensors antenna appears at the output of the WDM
Ph-ADC compressed by a factor of 3.4 - the time
stretching factor that is being considered. Further in-
vestigation showed that the level of the side lobes of
the spectrum of the compressed signal in the WDM
architecture is similar to the one obtained in the TDM
architecture as it is mainly dependent only on the
EOM (and the same EOM is used for both WDM and
TDM approaches).
5 DISCUSSION ON THE WDM
PH-ADC ARCHITECTURE
PERFORMANCE
In this section, the performance comparison between
the WDM and TDM Ph-ADC architectures is pre-
sented. Particularly, the signal-to-noise ratio (SNR)
evaluated from the approach presented in (Alves and
Cartaxo, 2011) and the degradation due to the fiber
non-linearities are analyzed.
5.1 SNR
In order to compare the SNR of the WDM Ph-ADC
architecture with its TDM version, let’s consider that
the optical peak power of each optical pulsed chan-
nel launched into the fiber in the WDM architecture
is identical to the one of the TDM architecture and
that the lengths of the two spools of fiber are the same
in both architectures. In addition, let’s consider also
that the propagation in both spools of fiber can be
well described as linear transmission. Within these
assumptions and taking into account that the photonic
structure of the WDM architecture is identical to the
one of the TDM architecture, it can be concluded that,
if the same electrical and optical gain levels are con-
sidered for both architectures, and that the insertion
losses imposed by the MUXs/DEMUXs can be ne-
glected, the SNR of the received signal of each branch
of the WDM architecture is identical to the SNR ob-
tained for the time stretched signal at the output of
the TDM architecture. However, the insertion losses
of the MUXs/DEMUXs are usually of the order of a
few dB and may impose some changes on the SNR
levels obtained in the WDM architecture.
In order to assess the SNR levels obtained by
both architectures, the peak SNR (Alves and Cartaxo,
2011) was evaluated for three different gain sets and
considering ideal MUXs/DEMUXs (without insertion
losses) and actual MUXs/DEMUXs (with insertion
losses of 5 dB). The three gain sets represent situa-
tions where the total noise variance is dominantly im-
paired by the noise introduced by the electrical trans-
mitter, by the optical amplifier or by the electrical re-
ceiver. In addition, the optical filter required by the
TDM architecture was modeled by a Gaussian shape
with a -3 dB bandwidth of 400 GHz (identical to the
one of the DEMUXs/MUXs of the WDM architec-
ture) in order to provide a fair comparison between
the results. It should be stressed that, in order to have
a fair comparison, also the same peak power for each
optical pulsed channel launched into the fiber in the
WDM architecture and in the TDM architecture has
been considered. This means that the insertion losses
of the AWG and of the MUX located at the input of
the first spool of fiber are not relevant for the analysis.
Table 1 shows the peak SNR levels obtained for
three cases: the WDM Ph-ADC considering the ab-
sence of MUXs/DEMUXs insertion losses, the WDM
Ph-ADC considering MUXs/DEMUXs with inser-
tion losses of 5 dB and the TDM Ph-ADC archi-
tecture. The results presented in Table 1 confirm
that both architectures provide the same SNR perfor-
mance since the system parameters are identical and
MUXs/DEMUXs with negligible insertion losses are
considered. However, when actual MUXs/DEMUXs
are considered, the peak SNR obtained in the WDM
architecture is lower than the one obtained for the
TDM case. This is due to the influence of the inser-
tion losses of the MUXs/DEMUXs on the power of
the received signal and on the noise variance. In or-
der to clarify this effect, let’s analyze separately the
influence of the insertion losses on each one of the
three different cases of gain sets.
• Total Noise Variance Dominantly Impaired
by the Noise of the Electrical Transmitter
(G
e
=40 dB, G
o
=30 dB, G
r
=50 dB). The total
noise variance is reduced due to the insertion
losses of the MUX and DEMUXs that are lo-
cated at the input and at the output of the second
spool of fiber, respectively. However, the signal
power is further decreased by the insertion losses
of the DEMUX located after the optical amplifier.
Therefore, the SNR obtained for the WDM archi-
tecture is lower than for the TDM.
• Total Noise Variance Dominantly Impaired by
the Noise of the Optical Amplifier (G
e
=20 dB,
G
o
=40 dB, G
r
=50 dB). In this case, the total noise
OPTICS 2011 - International Conference on Optical Communication Systems
118