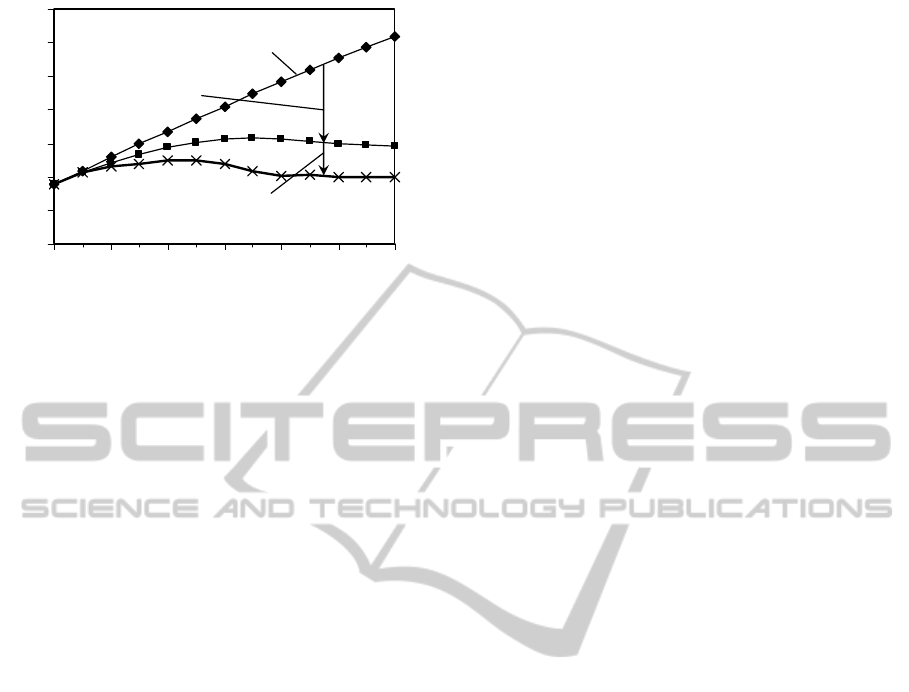
0
1
2
3
4
5
6
7
2005 2025 2045 2065 2085 2105 212
Year
Rise in radiative forcing (W/m
2
)
No climate control
CO
2
emissions
reduction
Solar radiation management
Figure 5: Contribution of CO
2
emissions reduction and
SRM to the mitigation of radiative forcing, W/m
2
relative
to 1900, in the case of stratospheric aerosol injection.
4 CONCLUDING REMARKS
SRM geoengineering is expected to be a lower-cost
option of climate control compared to CO
2
emissions reduction, and may considerably
contribute to the cost-effectiveness of global
climatic change mitigation. However, this option is
accompanied by the risk of rapid global warming if
the implementation of SRM is unexpectedly
terminated for any reason. As a guideline for the use
of SRM to avoid the risk, this study suggests that the
adverse effect should be controlled within an
acceptable range in case of unexpected SRM
termination at any time after its implementation. We
incorporated the guideline into the integrated
climate-economy model DICE by extending the
model and quantitatively showed the contributions
of CO
2
emissions reduction and SRM recommended
to prevent global warming.
The extension of the model brings increases in
the numbers of variables and constraint equations,
resulting in a longer computation time to solve the
model. The model is still solved within a minute
using a PC because it incorporates a very simplified
climate module; if we further extend the model to
deal with geographic distribution of climate change,
the computation time is estimated to increase, which
may impose a barrier to practical evaluation.
Finally, it should be emphasized that there are
some risks with the use of SRM other than those
considered in the present modeling study. The
quantitative results obtained from this study should
be interpreted as the economic potential of SRM use
assuming that such risks are low. If we needed to
regard these risks as considerably high, more
restrained use of SRM would be recommended.
REFERENCES
Brooke, A., Kendrick, D., Meeraus, A., 1992. GAMS: A
User's Guide, release 2.25, The Scientific Press, San
Francisco.
Brovkin, V., Petoukhov, V., Claussen, M., Bauer, E.,
Archer, D., Jaeger, C., 2009. Geoengineering climate
by stratospheric sulfur injections. Climatic Change,
92(3-4), 243-259.
Drud, A. S., 1994. CONOPT: A large-scale GRG code.
ORSA Journal on Computing, 6(2), 207-216.
German Advisory Council on Global Change (WBGU),
2003. Climate Protection Strategies for the 21st
Century: Kyoto and Beyond, WBGU, Berlin.
Hertzfeld, H. R., Williamson, R. A., Peter, N., 2005.
Launch Vehicles: An Economic Perspective, George
Washington University Space Policy Institute.
Washington, DC.
Kosugi, T., 2010. Role of sunshades in space as a climate
control option. Acta Astronautica, 67(1-2), 241-253.
Lenton, T., Vaughan, N. E., 2009. The radiative forcing
potential of different climate geoengineering options,
Atmospheric Chemistry and Physics, 9(15), 5539-5561.
McClellan, J., Sisco, J., Suarez, B., Keogh, G., 2010.
Geoengineering Cost Analysis: Final Report, UC01-
001; AR10-182, Aurora Flight Sciences Corporation.
Cambridge, MA.
Nordhaus, W. D., 2008. A Question of Balance: Weighing
the Options on Global Warming Policies, Yale
University Press. New Haven, CT.
Pearson, J., Oldson, J., Levin, E., 2006. Earth rings for
planetary environment control. Acta Astronautica,
58(1), 44-57.
The Royal Society, 2009. Geoengineering the Climate:
Science, Governance and Uncertainty, available via
http://royalsociety.org/geoengineeringclimate/.
Wigley, T. M. L., 2006. A combined mitigation/
geoengineering approach to climate stabilization.
Science, 314, 452–454.
SIMULTECH 2011 - 1st International Conference on Simulation and Modeling Methodologies, Technologies and
Applications
320