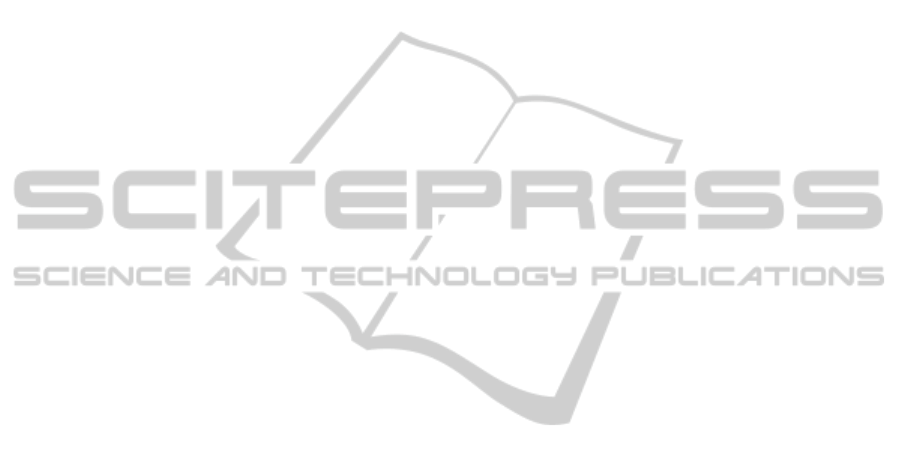
few attempts to model sludge minimization at source
within activated sludge process have been made
(Musser & Parker 2009; Camacho et al. 2005). In
this study, an Activated Sludge Model n°1 (ASM1)
(Henze et al. 2000) based model of sludge
minimization by thermal treatment is proposed and
validated against experimental data obtained during
a pilot study. The model is subsequently used to
investigate several scenarios and process
configurations for performance optimization.
2 METHODS
2.1 Pilots
Two identical lab-scale activated sludge processes
were built and conducted in parallel. One was used
as a control and the other included a thermal
disintegration step on the sludge recirculation line.
The volumes of rectangular aeration tanks and
settlers were 13.75 L and 8.3 L respectively. Bubble
diffusers located at the bottom of the reactors
provided aeration. In the combined system, thermal
treatment reactor was continuously included in the
recirculation line. Thermal treatment reactor
consisted of a stirred 900 mL glass recipient where a
heater (1 000 W) was dipped. Temperature was
measured by a Pt 100 probe and controlled by means
of a on/off temperature regulator.
2.2 Experimental Conditions
The pilots were continuously fed with primary
treated wastewater collected each week at the
Limoges (France) municipal wastewater treatment
plant. This plant has a 285 000 inhabitant-equivalent
capacity. Wastewater was kept in a refrigerated
(6°C) and agitated tank where it was directly
pumped to feed the pilots. Average characteristics of
feed wastewater during the experiments were: total
COD: 790 ± 140 mg O
2
.L
-1
, total suspended solids
(TSS): 370 ± 100 mg.L
-1
. Influent flow rate was set
up at 21.6 L.d
-1
corresponding to an influent
hydraulic residence time of 15.3 h in the aeration
tank. Sludge recirculation from the clarifier was
maintained at 100% of feed flow rate, allowing a 60
min return activated sludge retention time in the
thermal treatment reactor.
Aeration was operated by repeated
aerobic/anoxic cycles (2h/2h) in order to ensure
nitrification and denitrification. Air flow rate was
adjusted daily in order to have a dissolved oxygen
concentration around 2-4 mg.L
-1
in the reactors
during aerated phases (no limitation by oxygen
concentration). Solids residence time (i.e. sludge
age) was maintained at 15 days throughout the
experiments: settled sludge was wasted daily
accordingly considering sludge losses through the
effluent. The settlers were not considered in sludge
age calculations. A preliminary study indicated an
equal sludge production in the two processes when
thermal treatment was not carried out. The thermal
treatment experiment lasted 71 days.
Thermal treatment temperature was fixed at
90°C. The thermal sludge fraction (F) is defined here
as the fraction of sludge contained in aeration tank
treated each day. It was fixed at 0.2 d
-1
. The daily
duration of heater operation was calculated
accordingly. In order to avoid the injection of warm
sludge in the aeration tank, return activated sludge
was cooled at the outlet of the thermal reactor: the
pipe was circulated within fresh water cooled down
using a cryostat.
2.3 Calculation Procedure
The daily sludge production was calculated as grams
of Volatile Suspended Solids (VSS) produced. It is
based on a mass balance taking into account the
quantity of wasted sludge, the accumulation within
the reactor, and the sludge losses in the treated
effluent. As sludge production is directly related to
the amount of removed pollution, the sludge
production yield was calculated as the ratio of the
cumulative sludge production to the cumulative
COD removal in the reactors. This last calculation
considered a mass balance over COD entering and
leaving the system, neglecting the solubilized
COD during the treatment: the resulting sludge
production was therefore linked to global pollution
removal.
2.4 Model Development and
Calibration
Experimental data including influent, effluent,
activated and return activated sludge were grabbed
during whole experiment duration. They were
validated through the systematic procedure set up by
IWA Task Group on Good Modelling Practice
(2011).
2.4.1 Influent Wastewater Characterization
Fractionation of influent wastewater was deduced
from experimental measurements (influent and
effluent total and dissolved COD as well as TSS,
SLUDGE MINIMIZATION BY THERMAL LYSIS DURING BIOLOGICAL WASTEWATER TREATMENT -
Experimental Study, Model Set Up and Calibration
361