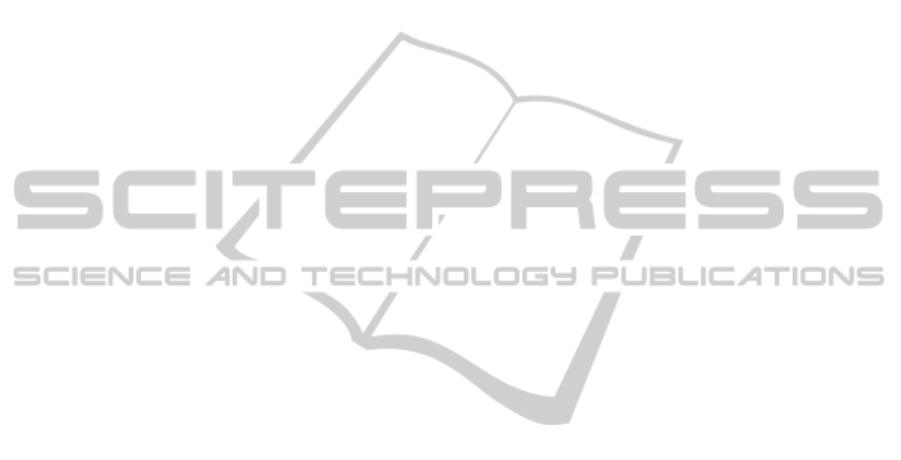
Long But Promising Route” Sensors, 10(12) 11530-
11555; doi:10.3390/s101211530.
Almeida, M. G., Macieira, S., Gonçalves, L. L., Huber, R.,
Cunha, C. A., Romão, M. J., Costa, C., Lampreia, J.,
Moura, J. J. G., Moura I., 2003. “The Isolation and
characterization of Cytochrome c Nitrite Reductase
Subunits (NrfA and NrfH) from Desulfovibrio
desulfuricans ATCC 27774. Re-evaluation of the
spectroscopic data and redox properties”, Eur. J.
Biochem. 270, 3904-3915.
Almeida, M. G., Silveira, C. M., Moura, J. J. G., 2007.
“Biosensing Nitrite Using the System Nitrite
Redutase/Nafion/Methyl Viologen - A Voltammetric
Study” Biosens. Bioelectron. 22, 2485-2492.
Bryan, N. S., Fernandez, B. O., Bauer, S. M., Garcia-
Saura, M. F., Milsom, A. B., Rassaf, T., Maloney, R.
E., Bharti, A., Rodriguez, J., Feelisch, M., 2005.
“Nitrite is a signaling molecule and regulator of gene
expression in mammalian tissues” Nat. Chem. Biol. 1,
290-297.
Chen, H., Mousty, C., Cosnier, S., Silveira, C. Moura, J. J.
G., Almeida, M. G., 2007. “Highly Sensitive ccNiR
Biosensor Electrical-Wired by [ZnCrAQS
2
] for Nitrite
Determination” Electrochem. Comm. 9, 2240-2245.
da Silva, S., Cosnier, S., Almeida, M. G., Moura, J. J. G.,
2004. “An Efficient Poly(pyrrole)-Nitrite Reductase
Biosensor for the Mediated Detection of Nitrite”
Electrochem. Comm. 6, 404-408.
Ellis, G., Adatia, I., Yazdanpanah, M., Makela, S. K,
1998. “Nitrite and nitrate analyses: A clinical bioche-
mistry perspective”. Clin. Biochem. 31, 195-220.
Hord, N. G., Tang, Y., Bryan N. S., 2009. “Food sources
of nitrates and nitrites: The physiologic context for
potential health benefits” Am. J. Clin. Nutr. 90, 1-10.
Jubete, E., Loaiza, O. A., Ochoteco, E., Pomposo, J. A.,
Grande, H., Rodrıguez, J., 2009. “Nanotechnology: A
Tool for Improved Performance on Electrochemical
Screen-Printed (Bio)Sensors” J. Sensors, Article ID
842575, 13 pages, doi:10.1155/2009/842575.
Lundberg, J. O. et al., 2009. “Nitrate and nitrite in biology,
nutrition and therapeutics” Nature, 12, 865-869.
Ochoteco, E., Jubete, E., Pomposo, J. A., Grande, H. et al.
2009 Patent Application Number E200930539.
Silveira, C. M., Gomes, S. P., Araújo, A. N., Couto, C. M.
C. M., Montenegro, M. C. B. S. M., Silva, R., Viana,
A. S., Todorovic, S., Moura, J. J. G., Almeida, M. G.,
2010a. “An Efficient Mediatorless Biosensor for
Nitrite Determination” Biosens. Bioelectron. 25, 2026-
2032.
Silveira, C., Baur, J., Cosnier, S., Moura, J. J. G.,
Holzinger, M., Cosnier, S., Almeida, M. G., 2010b.
"Enhanced direct electron transfer of a multihemic
nitrite reductase on SWCNT modified electrodes"
Electroanalysis, 22, 2973–2978.
Silveira, C. M., Besson, S., Moura, I., Moura, J. J. G.,
Almeida, M. G., 2010c. ”Measuring the Cytochrome c
Nitrite Reductase Activity – Practical considerations
on the enzyme assays”, Bioinorg. Chem. Applic.,
Volume 2010, Article ID 634597, 8 pages,
doi:10.1155/2010/634597
Zhang, Z., Xia, S., Leonard, D., Jaffrezic-Renault, N.,
Zhang, J., Bessueille, F., Goepfert, Y. S, Wang, X.,
Chen, L., Zhu, Z., Zhao, J., Almeida, M. G., Silveira,
C. M., 2009. “A novel nitrite biosensor based on
conductometric electrode modified with cytochrome c
nitrite reductase composite membrane” Biosens.
Bioelectron. 24, 1574–1579.
BIODEVICES 2012 - International Conference on Biomedical Electronics and Devices
36