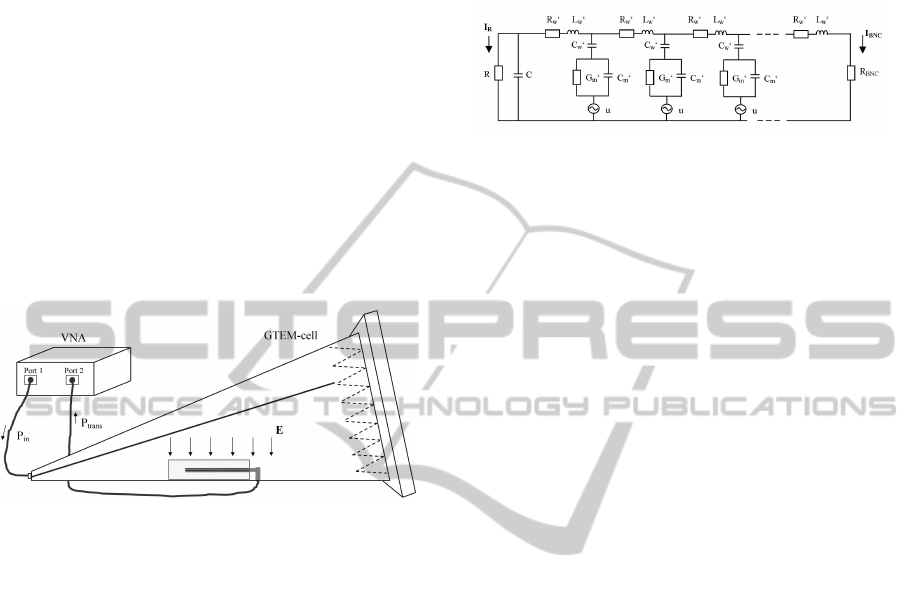
was connected to a GTEM cell (Model 5402, ETS-
Lindgren, USA) as it is shown in Figure 1. The
output power of the VNA from port 1 was used to
generate a homogeneous transverse electromagnetic
field inside the GTEM cell. The electrode was posi-
tioned inside a medium and connected to port 2 via a
BNC-connector at one end and left in contact to the
medium on the other end. Several different media
were investigated: air, silicone and a phantom mus-
cle. The latter was a mixture of the gelling agent
TX151 (Brunschwig Chemie, Netherlands), distilled
water and sodium chloride (Chou et al., 1995). The
electrode axis was in a horizontal orientation and thus
perpendicular to the electric field, which is vertically
aligned. The electric field was measured with an elec-
tric field probe (D.A.R.E.!! Instruments, RadiSense,
LP1001A, Netherlands).
Figure 1: Connection of the VNA and the GTEM cell.
The electrode under test is horizontally positioned and sur-
rounded by a medium.
The Network Analyser measures the amplitude
and phase of the four scattering parameters in a fre-
quency range from 3 MHz to 300 MHz. Only the
reverse (S
12
) or forward (S
21
) power gain – both are
equal (reciprocity theorem) – are relevant in our in-
vestigation. The S
21
-parameter is defined as the ratio
of the transmitted power at the matched port 2 to the
input power at port 1. We compared the amplitudes
of the S
21
-parameter of different electrode geometries
(straight wires, completely and partially solenoidal
wires) over the complete frequency range in air and
silicone and at 64 MHz and 128 MHz in the phantom
material, which are the RF frequencies for the 1.5 T
and 3 T MRI-scanners, respectively.
The electrodes tested in viscous materials (e.g. sil-
icone) were moulded into these materials to provide a
perfect contact between the tip of the electrode and
the medium.
2.2 Transmission Line Theory
A part of the equivalent circuit of the electrode be-
ing excited by the electric field of the GTEM cell is
shown in figure 2. It is important to note that a the-
oretical description of the electrode as a transmission
line must include environmental parameters in addi-
tion to intrinsic parameters of the electrode (Konings
et al., 2000). In the presented setup, the electrode is
Figure 2: The equivalent circuit of the electrode in a per-
pendicular electric field inside the GTEM cell.
considered to be the inner conductor and the housing
of the GTEM cell the outer conductor of the trans-
mission line. The field perpendicular to the electrode
axis induces a voltage u between the electrode and
the GTEM housing. The equivalent circuit is a cas-
cade connection of n consecutive quadripoles (three
of them are shown in figure 2). Each quadripole
is characterized by two complex impedances, one
along the wire, consisting of a resistance R
0
w
in se-
ries with an inductance L
0
w
, another between the in-
ner and outer conductor, consisting of C
0
w
in series
with a parallel connection of G
0
m
and C
0
m
. R
0
w
and
L
0
w
are the resistance and inductance of the wire per
quadripole length, respectively, C
0
w
is the capacitance
along the insulation layer between wire and medium
per quadripole length, G
0
m
and C
0
m
are the conductance
and capacitance between the surface of the insulation
and the outer conductor per quadripole length. R
BNC
is the resistance of the BNC-connection (=50Ω). For
simplicity, the lead tip is described as a hemisphere,
with surface area of the lead tip’s real contact area,
and thus representing the capacitance C of a hemi-
sphere and an analogous resistance R. The total resis-
tance and capacitance of the wire were also calculated
by geometric considerations and checked by low fre-
quency measurements and then divided by the num-
ber of quadripoles to yield the values per quadripole
length. A calculation of R
0
w
and C
0
w
is trivial for any
wire with concentric insulation. L
0
w
was estimated via
well known inductance formulas for solenoids and
straight wires. To determine G
0
m
and C
0
m
we consid-
ered the transmission line to be a coaxial line, with the
radius of the outer conductor being equal to the dis-
tance between electrode and cell housing, while the
radius of the inner conductor is equal to the wire ra-
dius for straight wires and equal to the coil radius for
solenoid wires.
The equivalent circuit is a linear network with n
voltage sources and described by 3n+2 independent
first-order differential equations. They can be written
in state space representation:
BIODEVICES 2012 - International Conference on Biomedical Electronics and Devices
316