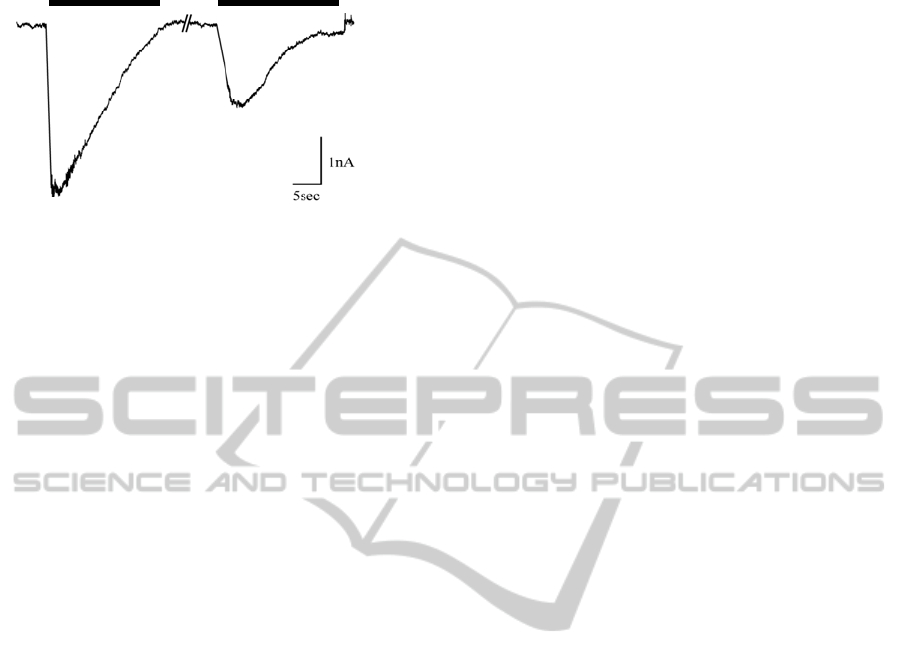
CAP
Figure 7: Whole cell channel current record of TRPVI
expressed on HEK293 cell by capsaicin stimulations
measured by ion channel biosensor based on the
incubation type planar patch clamp method.
4 CONCLUSIONS
Ion channel biosensor based on the incubation type
planar patch clamp method was developed and the
basic properties were investigated. Due to the
existence of ECM protein at the cleft between the
cell membrane and the substrate surface near the
miropore, it is not easy to realize the high seal
resistance (giga-ohm seal). In the present case using
collagen 4 as ECM, the seal resistance was usually
about 10 MΩ, and the noise level was 7 pA with the
1 kHz low pass filter. The main noise sources were
excess current noise and the thermal noise generated
at micro pore resistance (R
a
) and the seal resistance
(R
j
). All these noises can be reduced by increasing
the seal resistance. Operation of the light-gated ion
channel, ChRWR, was investigated by the
incubation type planar patch clamp method using
laser (λ = 473 nm) stimulations. The channel
current profile and its membrane potential
dependence well agreed to the reported data
measured by pipette patch clamp method. So we
think that light-gated method is also useful in the
neural network function analysis and high
throughput screening application based on the
incubation type planar patch clamp method, and also
useful in the simple performance check of these
devices. The biosensor operation was examined
using TRPV1-expressing HEK293 cells. Quite high
sensitivity was confirmed. But for the single channel
recording, more than several times improvement of
the seal resistance is required.
ACKNOWLEDGEMENTS
We appreciate Dr. Hitoshi OHMORI and Mr.
Yosuke HACHISU at RIKEN for their support in
making brass mold by ultra-precision machining
equipment.
REFERENCES
Bayley, H., Cremer, P.S., 2001. Nature 413, 226.
Caterina, M. J., Schumacher, M. A., Tominaga, M.,
Rosen, T. A., Levine, J. D., Julius, D., 1997. Nature
389, 816.
Erickson, J., Tooker, A., Tai, Y. C., Pine, J., 2008.,
Journal of Neuroscience Methods 175, 1.
Fertig, N., Blick, R. H., Behrends, J. C., 2002. Biophys. J.
82,3056.
Li, X. H., Klemic, K. G., Reed, M. A., Fred, J., Sigworth,
F. J. 2006. Nano Letters 6 , 815.
Matthews, B., Jack, W., 2006. J. Microelectromech. 15
214.
Mayer, M., Kriebel, J. K., Tosteson, M. T., Whitesides, G.
M., 2003. Biophysical J. 85, 2684.
Pantoja, R., Nagarah, J. M., Starace, D. M., Melosh, N. A.
Blunck, R., Bezanilla, F., Heath, J. R., 2004. Biosens.
Bioelectron. 20, 509.
Petreanu, L., Huber, D., Sobczyk, A., Svoboda, K., 2007,
Nature Neurosci. 10, 663.
Reska, A., Gasteier, P., Schulte, P., Moeller, M.,
Offenhäusser, A., Groll, J., 2008. Advanced Materials
20,2751.
Sett, A, Burkhardt, C., Weber, U., Stiphout, P.V., Knott,
T. 2003. Receptors and Channels 9, 59.
Sordel, T., Garnier-Raveaud, S., Sauter, F., Pudda, C., F.
Marcel, F., Waard, M. D., Arnoult, C., Vivaudou, M.,
Chatelain, F., Picollet-D’hahan, N., 2006. J.
Biotechnol. 125, 142.
Tao, H. W., Zhang, L. I., Bi, G., Poo, M., 2000. J.
Neurosci. 20, 3233.
Taylor, A. M., Dieterich, D. C., Ito, H. T., Kim, S. A.,
Schuman, E. M. 2010. Neuron 66, 57.
BIODEVICES 2012 - International Conference on Biomedical Electronics and Devices
148