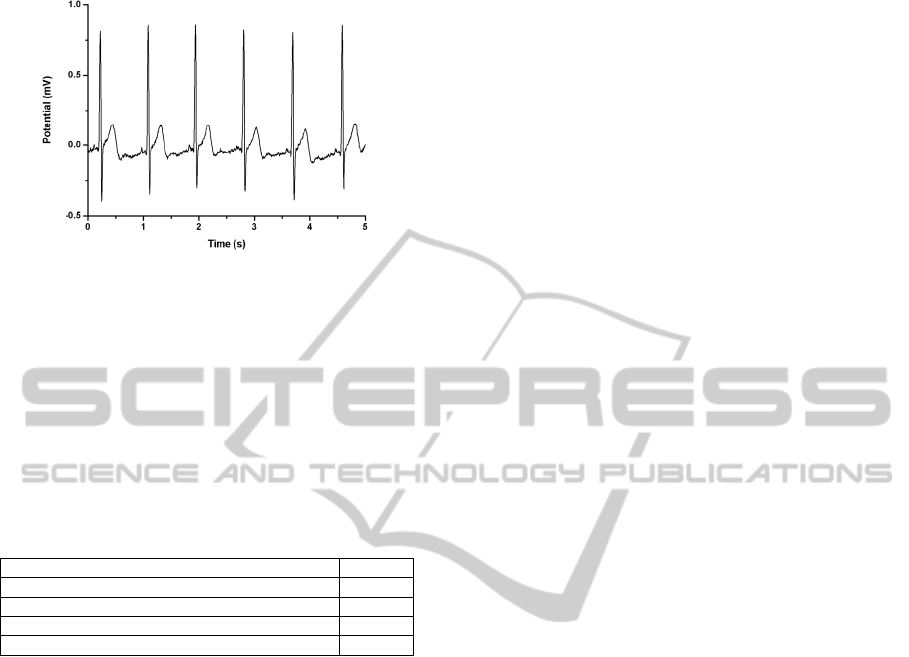
number of communication errors occurred over time.
Figure 3: ECG lead signal acquired by the developed
wearable sensor.
Table 2 shows a list of different statistics obtained
during a testing period of one hour, within which the
3 wireless sensor nodes were performing signal
acquisition and transmitted their monitored data
when requested by the network coordinating node,
following a TDMA communication scheme.
Table 2: Statistics of error performance in wireless
communications within the developed network, during a
testing period of one hour.
Connection losses 2
Packets lost due to link errors 60
Duplicate packets received 10
Total number of generated packets/TDMA slots 1383
Packet error rate 5.1%
The obtained packet rate of 5.1% is considerable,
although it could be acceptable for continuous long-
term monitoring applications, where loss of data is
less critical.
5 CONCLUSIONS
From a preliminary analysis of the developed
system, it is expected that the use of a wireless
network of single-lead ECG sensors, using a
protocol based on TDMA and operating in a less
crowed band, could provide reliable support for
continuous monitoring of independent single-lead
signals and for continuous 12-lead ECG synthesis.
Future work will involve testing of the accuracy
of the proposed system for 12-lead ECG synthesis in
volunteers and the development of a new
miniaturised version of the single-lead ECG sensors.
Moreover, tests should be performed to evaluate up
to what degree the data losses caused by errors in
wireless communications affect the accuracy and the
performance of the system for continuous
monitoring of a synthesized 12-lead ECG.
ACKNOWLEDGEMENTS
This work was supported by the Portuguese
Foundation for Science and Technology under grant
SFRH/BD/40341/2007.
REFERENCES
Atoui, H., Fayn, J., Rubel, P., 2010. A Novel Neural-
Network Model for Deriving Standard 12-Lead ECGs
From Serial Three-Lead ECGs: Application to Self-
Care. IEEE Trans. Inf. Technol. Biomed., 14(3),
883-890.
Dower, G. E., Yakush, A., Nazzal, S. B., Jutzy, R. V.,
Ruiz, C. E., 1988. Deriving the 12-lead
electrocardiogram from four (EASI) electrodes. J.
Electrocardiol., 21, 182-187.
Figueiredo, C. P., Becher, K., Hoffmann, K.-P., Mendes,
P. M., 2010. Low Power Wireless Acquisition Module
for Wearable Health Monitoring Systems. Proc. 32nd
Ann. Int. IEEE EMBS Conf., 704-707.
Hao, Y., Foster, R., 2008. Wireless body sensor networks
for health-monitoring applications. Physiol. Meas., 29,
R27-R56.
Klootwijk, P. et al., 1998. Comparison of usefulness of
computer assisted continuous 48-h 3-lead with 12-lead
ECG ischaemia monitoring for detection and
quantitation of ischaemia in patients with unstable
angina. Eur. Heart J., 18(6), 931-940.
Milenković, A., Otto, C., Jovanov, E., 2006. Wireless
sensor networks for personal health monitoring: Issues
and an implementation. Comp. Commun., 29,
2521-2533.
Nelwan, S. P., Kors, J. A., Meij, S. H., Bemmel, J. H. v.,
Simoons, M.L., 2004. Reconstruction of the 12-lead
ECG from Reduced Lead Sets. J.Electrocardiol., 37,
11-18.
Nield, I., Heatley, D. T. J., Kalawsky, R. S., Bowmann, P.
A., 2004. Sensors Networks for Continuous Health
Monitoring. BT Technol. J., 22(3), 130-139.
Omeni, O. C., Eljamaly, O., Burdett, A. J., 2007. Energy
Efficient Medium Access Protocol for Wireless
Medical Body Area Sensor Networks. Proc. 4th IEEE-
EMBS Int. Summer School & Symp. Medical Devices
and Biosensors, 29-32.
Pantepoulos, A., Bourbakis, N. G., 2010. A Survey on
Wearable Sensor-Based Systems for Health
Monitoring and Prognosis. IEEE Trans. Syst., Man,
Cybern. C, Appl. Rev., 40(1), 1-12.
Steltenkamp, S., Becher, K., Doerge, T., Ruff, R.,
Hoffmann, K.-P., 2009. Electrode structures for
acquisition and neural stimulation controlling the
cardiovascular system. Proc. 31st Ann. Int. IEEE
EMBS Conf., 5478-5481.
Trobec, R., Tomašić, I., 2011. Synthesis of the 12-Lead
Electrocardiogram From Differential Leads. IEEE
Trans. Inf. Technol. Biomed., 15(4), 615-621.
BIODEVICES 2012 - International Conference on Biomedical Electronics and Devices
332