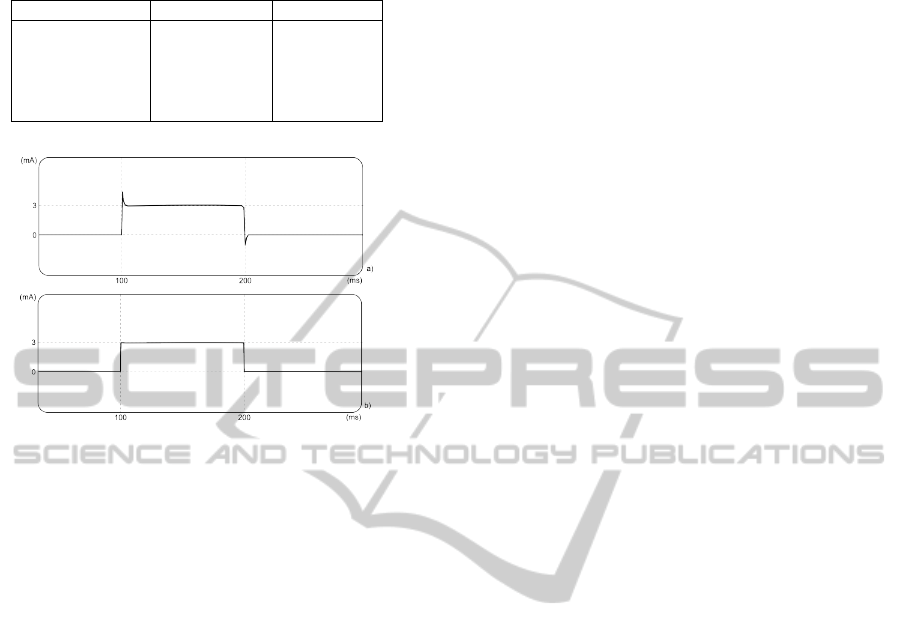
Table 2: Voltage variation vs load variation in constant volt-
age mode.
Resistance (kΩ) Current (mA) Tension (V)
1.80 1.67 3.01
1.50 2.00 2.97
1.31 2.30 3.05
1.19 2.53 3.01
0.82 3.64 2.93
Figure 6: Effect of the skin nonlinear dynamics represented
by the C1 capacitor: a) With C1=1µF; b) Without C1.
we report the results obtained in the constant voltage
mode.
As it was expected, in constant current mode the
voltage varies to equalize the variation in the resis-
tance, keeping the applied current constant.
The same effect, but now applied in the current is
verified in the constant voltage mode were the current
compensate the resistance variations keeping the ap-
plied voltage in the desired value. In constant voltage
mode, the system can’t keep the voltage regulation for
load values bellow 750Ω, because the system is cur-
rently limited to 4mA.
The implementation of this model enabled the
evaluation of the effect of the skin impedance non-
linear dynamics. For values of C1 higher than 1 µF
we observed an overshooting effect which can exceed
about 20% the programmed current value. This effect
is exposed in Figure 6, which shows the signal of cur-
rent corresponding to a square wave of 2Hz and 3mA
of amplitude with and without the C1 effect.
With this study we evaluated the constant current
and constant voltage mode and guaranteed the correct
operation of the developed device.
4 CONCLUSIONS
The main contributions of this work are related with
the high flexibility in the control of the stimulation
session in association with the portability and low di-
mensions of the device.
With this device is possible to automate a stimu-
lation session and change it in real time, allowing, for
example, an external evaluator to analyse the differ-
ences in gait according to the protocol that is applied.
The high portability of this system and its user-
friendly characteristics may allow its usage in a pa-
tient home through ambient assisted living with a real-
time protocol controlled in a web based environment
by the physician.
5 FUTURE WORK
As a continuation of this work, we are now testing the
device in experimental animals, with good results to
the date.
We are also implementing a stimuli wave form
control, which will enable the study of the effect of
different wave forms for stimuli application in the
muscular structures.
Another advance to this work resides with the
adaptation of the hardware’s amplification module for
surface electrostimulation application.
REFERENCES
Bajd, T., Kralj, A., Stefancic, M., and Lavrac, N. (1999).
Use of functional electrical stimulation in the lower
extremities of incomplete spinal cord injured patients.
In Artif Organs, 23(5):403-9.
Cheng, K., Lu, Y., Tong, K., Rad, A., Chow, D., and Su-
tanto, D. (2004). Development of a circuit for func-
tional electrical stimulation. In IEEE Transactions
on Neural Systems and Rehabilitation Engineering,
vol.12, no.1.
Dorgan, S. and Lake, R. (1999). A model for human skin
impedance during surface functional neuromuscular
stimulation. In IEEE Transactions on Rehabilitation
Engineering, vol. 7, no. 3.
Lake, D. (1992). Neuromuscular electrical stimulation. an
overview and its application in the treatment of sports
injuries. In Sports Med 13 320-36.
PLUX (2011). PLUX - Wireless Biosignals S.A.
www.plux.info.
Robertson, V., Ward, A., Low, J., and Reed, A. (2006).
Electrotherapy Explained: Principles and Practice.
Elsevier Health Sciences, 4th edition.
Thrasher, T. and Popovic, M. (1999). Funcional electrical
stimulation of walking: Function, exercise and reha-
bilitation. In Ann Readapt Med Phys. 51(6):452-60.
Zhang, D., Guan, T., Widjaja, F., and Ang, W. (2007). Func-
tional electrical stimulation in rehabilitation engineer-
ing: A survey. In Proceedings of the 1st international
convention on Rehabilitation engineering and assis-
tive technology.
BIODEVICES 2012 - International Conference on Biomedical Electronics and Devices
340