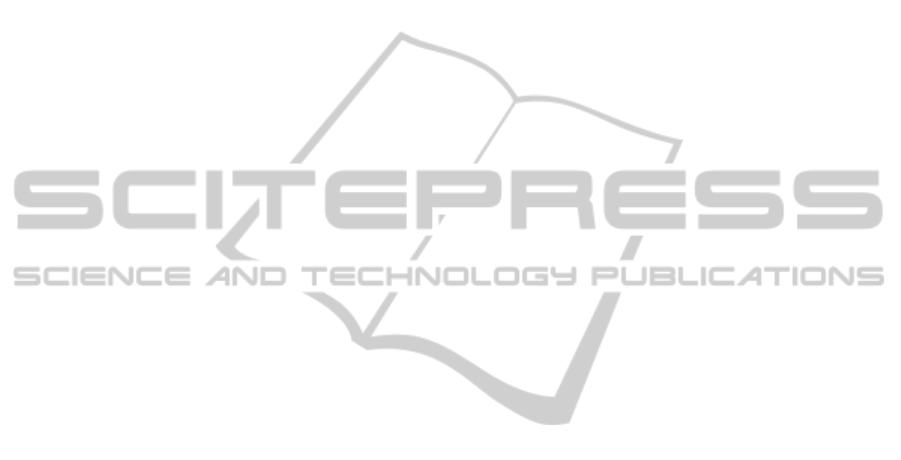
This BP study allowed us to conclude that the cus-
tomized calibration (Calibration 1) provides better re-
sults than the general (Calibration 2) for the tests per-
formed in this work. Still, it can be assumed that with
both calibrations was possible to obtain the SBP and
DBP.
4 CONCLUSIONS
The main goal of the present work was to develop a
device that allows the measurement of both S
p
O
2
and
BP without using a cuff, in a non-invasive, confort-
able and continuous mode.
The results show that the device works using both
ECG and pulse oximeter, or using only an optical
technique with the placement of two sensors, one in
finger and other in wrist (with two pulse oximeters).
It was also concluded that for BP estimation, a cal-
ibration for each subject, as an inicial calibration of
sensor for each user, gives more accurate results than
the BP estimated from a general calibration, even with
an input parameter.
This study proved to be a starting point for the
development of this device, that after some improve-
ments, specialy in signal processing, will conduct to
a medical device.
5 FUTURE WORK
A more effective calibration of the pulse oximeter,
with more subjects and with an induced hypoxic state,
is an important improvement for the next step of the
oxiPlux development. The transition from offline to
real-time software is also a development that will be
done for this sensor.
For the BP study, all the used approaches recourse
to linear regessions to relate PTT with BP, but an al-
ternative approach could be made using non-linear re-
lations to relate that two parameters. The study and
application of other body correlation factors, related
to height, can be also usefull to obtain more accurate
results.
ACKNOWLEDGEMENTS
This work was partially supported by National Strate-
gic Reference Framework (NSRF-QREN) under
projects ”LUL”, ”Affective Mouse”, ”wiCardioResp”
and ”Do-IT”, and Seventh Framework Programme
(FP7) program under project ICT4Depression, whose
support the authors gratefully acknowledge.
REFERENCES
Daminani, F. R. (2010). Proposta de prot
´
otipo de um
ox
´
ımetro de pulso empregando tecnologia FPAA.
Master’s thesis, Universidade de S
˜
ao Paulo - Escola
de Engenharia de S
˜
ao Carlos, So Paulo.
Fung, P., Dumont, G., Ries, C., Mott, C., and Ansermino,
M. (2004). Continuous noninvasive blood pressure
measurement by pulse transit time. In Engineering
in Medicine and Biology Society, 2004. IEMBS’04.
26th Annual International Conference of the IEEE,
volume 1, pages 738–741. IEEE.
Gesche, H., Grosskurth, D., Kuchler, G., and Patzak, A.
(2011). Continuous blood pressure measurement by
using the pulse transit time: comparison to a cuff-
based method. European Journal of Applied Physi-
ology, pages 1–7.
Haahr, R. G. (2006). Reflectance Pulse Oximetry Sensor
for the Electronic Patch. Master’s thesis, Technical
University of Denmark - MIC - Department of Micro
and Nanotechnology, Denmark.
Naschitz, J., Bezobchuk, S., Mussafia-Priselac, R., Sundick,
S., Dreyfuss, D., Khorshidi, I., Karidis, A., Manor, H.,
Nagar, M., Peck, E., et al. (2004). Pulse transit time
by r-wave-gated infrared photoplethysmography: Re-
view of the literature and personal experience. Journal
of clinical monitoring and computing, 18(5):333–342.
Nonin (2011). Nonin - nonin medical inc. [online] avalil-
able at: http://www.nonin.com/ [accessed 5 september
2011].
Payne, R., Symeonides, C., Webb, D., and Maxwell, S.
(2006). Pulse transit time measured from the ecg:
an unreliable marker of beat-to-beat blood pressure.
Journal of Applied Physiology, 100(1):136.
PS (2011). Portal da saude - ministerio da saude [online]
avalilable at: http://www.portaldasaude.pt/ [accessed
5 september 2011].
Reddy, K., George, B., Mohan, N., and Kumar, V. (2009).
A Novel Calibration-Free Method of Measurement
of Oxygen Saturation in Arterial Blood. Instru-
mentation and Measurement, IEEE Transactions on,
58(5):1699–1705.
Reisner, A., Shaltis, P., McCombie, D., and Asada, H.
(2008). Utility of the photoplethysmogram in circu-
latory monitoring. Anesthesiology, 108(5):950.
Sola, A. et al. (2005). Pulse oximetry in neonatal care in
2005. A comprehensive state of the art review. In
Anales de Pediatr
´
ıa, volume 62, page 266.
WelchAllyn (2011). Welch allyn [online] avalilable
at: http://www.welchallyn.com/[accessed 5 september
2011].
WHO (2011). World health organization - the top
10 causes of death [online] - available at:
http://www.who.int/mediacentre/factsheets/fs310
/en/index.html [accessed 5 september 2011].
Wong, M., Poon, C., and Zhang, Y. (2009). An evalua-
tion of the cuffless blood pressure estimation based
on pulse transit time technique: a half year study on
normotensive subjects. Cardiovascular Engineering,
9(1):32–38.
BIODEVICES 2012 - International Conference on Biomedical Electronics and Devices
206