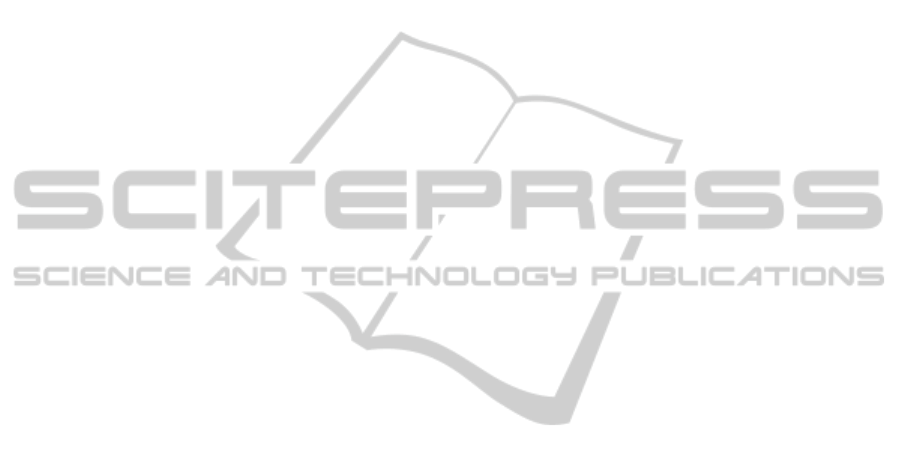
low value of β, corresponding to low disability. In the
same way, high disability correspond to higher track-
ing error, and lower disability correspond to a better
accuracy in the performance of the movement com-
pared with the healthy subject.
4 CONCLUSIONS
In this paper, in order to control an orthosis, an op-
timal approach is proposed to design a PI controller
according to disability of the patients. This disabil-
ity is simulated by means of physiological static opti-
mization approach where the muscular forces of SCI
are obtained in a process that combines the actuation
of the muscles and the external actuation provided by
the orthosis. Those forces are used to design a proper
controller for the external actuation. Considering pa-
tients with a high disability, the controller is tuned to
perform the movement so as to allow the patient to
achieve the movement but in a longer cycle compared
with patients with less disability, where the controller
is tuned giving priority to the accuracy of the move-
ment. Patients with less power in his muscle –high
disability–, need more time in a gate cycle to walk,
whereas patients with low disability need less time.
This idea is shown through some three types of dis-
ability, i.e. high disability, fair disability and low dis-
ability. The simulation results show the efficiency of
the proposed method.
ACKNOWLEDGEMENTS
This work was supported by the Spanish Ministry of
Science and Innovation under the project DPI2009-
13438-C03. The support is gratefully acknowledged.
REFERENCES
Ackermann, M. (2007). Dynamics and energetics of
walking with prostheses. PhD thesis, University of
Stuttgart.
Ackermann, M. and Schiehlen, W. (2006). Dynamic anal-
ysis of human gait disorder and metabolical cost esti-
mation. Archive of Applied Mechanics, 75:569–594.
Alonso, J., Romero, F., P
`
amies-Vil
`
a, R., Lugr
´
ıs, U., and
Font-Llagunes, J. (2011). A simple approach to esti-
mate muscle forces and orthosis actuation in powered
assisted walking of spinal cord-injured subjects. Proc.
EUROMECH Coll. 511 Biomechanics of Human Mo-
tion 2011, Ponta Delgada, Azores, Portugal.
Amankwah, K., Triolo, R., and Kirsch, R. (2004). Ef-
fects of spinal cord injury on lower-limb passive joint
moments revealed through a nonlinear viscoelastic
model. Journal of Rehabilitation Research & Devel-
opment, 41:15–32.
Dollar, A. and Herr, H. (2008). Lower extremity exoskele-
tons and active orthoses: challenges and state-of-the-
art. IEEE T Robotics, 24:1–15.
Edrich, T., Riener, R., and Quintern, J. (2000). Analysis of
passive elastic joint moments in paraplegics. IEEE
Transactions on Biomedical Engineering, 47:1058–
1065.
Filippi, P. (1942). Device for the automatic control of the
articulation of the knee applicable to a prosthesis of
the thigh.
Font-Llagunes, J., P
`
amies-Vil
`
a, R., Alonso, J., and Ur-
bano Lugr
´
ıs, U. (2011). Simulation and design of an
active orthosis for an incomplete spinal cord injured
subject. Procedia IUTAM, 2:68–81.
Gerritsen, K., van den Bogert, A., Hulliger, M., and Zer-
nicke, R. (1998). Intrinsicmuscle properties facilitate
locomotor control: a computer simulation study. Mo-
tor Control, 2.
HosseinNia, S., Romero, F., Vinagre, B., Alonso, F., Te-
jado, I., and Font-Llagunes, J. (2011). Hybrid model-
ing and fractional control of a sckafo orthosis for gait
assistance. In ASME 2011 International Design En-
gineering Technical Conferences and Computers and
Information in Engineering Conference.
Kao, P., Lewis, C., and Ferris, D. (2010). Invariant ankle
moment patterns when walking with and without a
robotic ankle exoskeleton. Journal of Biomechanics,
43:203–209.
Lebiedowska, M. and Fisk, J. (1999). Passive dynamics of
the knee joint in healthy children and children affected
by spastic paresis. Clinical Biomechanics, 14(9):653–
660.
Menegaldo, L., Fleury, A., and Weber, H. (2006). dd. Jour-
nal of Biomechanics, 39:1787–1795.
Silva, P. C., Silva, M. T., and Martins, J. M. (2010). Eval-
uation of the contact forces developed in the lower
limb/orthosis interface for comfort design. Multibody
System Dynamics, 24:367–388.
Thomas, C. and Grumbles, R. (2005). Muscle atrophy after
human spinal cord injury. Biocybernetics & Biomedi-
cal Engineering, 25:39–46.
Vukobratovic, M., Ciric, V., and Hristic, D. (1972). Contri-
bution to the study of active exo-skeletons. Proceed-
ings of the 5th IFAC Congress, Paris, France,.
Winter, D. (1991). Biomechanics and motor control of hu-
man gait: normal, elderly and pathological. Univer-
sity of Waterloo Press, 2nd edition.
Yamaguchi, G., Moran, D., and Si, J. (1995). A compu-
tationally efficient method for solving the redundant
problem in biomechanics. Journal of Biomechanics,
28:999–1005.
Zajac, F. (1989). Muscle and tendon: Properties, models,
scaling and applications to biomechanics and motor
control. Critical Reviews in Biomedical Engineering,
17:359–411.
BIODEVICES 2012 - International Conference on Biomedical Electronics and Devices
64