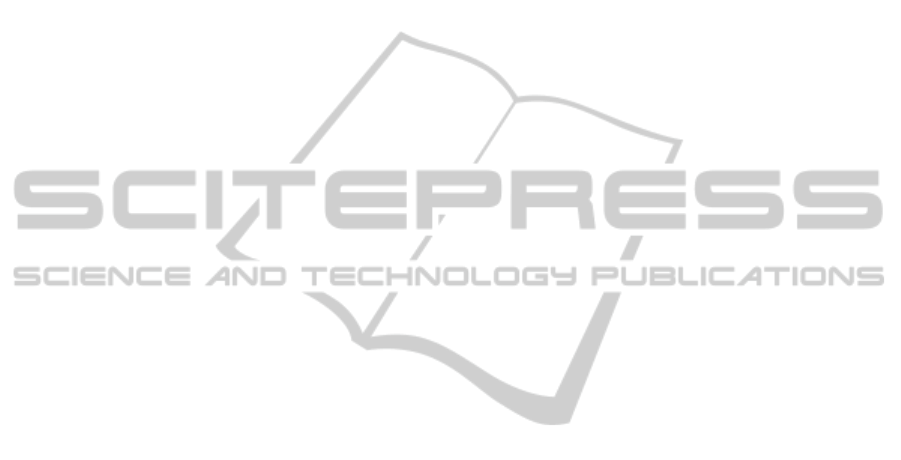
REFERENCES
Adler, A., Amyot, R., Guardo, R., Bates, J. H.,
Berthiaume, Y., 1997. Monitoring changes in lung air
and liquid volumes with electrical impedance
tomography. Journal of Applied Physiology. 83(5):
1762-7.
Braak, H., Braak, E., 2000. Pathoanatomy of Parkinson's
disease. Journal of Neurology. 247(2): II3-10.
Benabid, A. L., Pollak, P., Louveau, A., Henry, S., de
Rougemont, J., 1987. Combined (thalamotomy and
stimulation) stereotactic surgery of the VIM thalamic
nucleus for bilateral Parkinson disease. Applied
Neurophysiology. 50(1-6): 344-6.
Foster, K. R., Schwan, H. P., 1989. Dielectric properties of
tissues and biological materials: a critical review.
Critical reviews in biomedical engineering.
17(1): 25-104.
Gimsa, J., Habel, B., Schreiber, U., van Rienen, U.,
Strauss, U., Gimsa, U., 2005. Choosing electrodes for
deep brain stimulation experiments – electrochemical
considerations. Journal of Neuroscience Methods.
142(2): 251-65.
Gimsa, U., Schreiber, U., Habel, B., Flehr, J., van Rienen,
U., Gimsa, J., 2006. Matching geometry and
simulation parameters of electrodes for deep brain
stimulation experiments – numerical considerations.
Journal of Neuroscience Methods. 150(2): 212-27.
Grill, W. M., Mortimer, J.T., 1994. Electrical properties of
implant encapsulation tissue. Annals of Biomedical
Engineering. 22(1): 23-33.
Henning, J., 2007. Wirkungen der tiefen Hirnstimulation –
Analyse der Gen- und Proteinexpression in einem
optimierten Rattenmodell. Dissertation. University of
Rostock.
Harnack, D., Winter, C., Meissner, W., Reum, T., Kupsch,
A., Morgenstern, R., 2004. The effects of electrode
material, charge density and stimulation duration on
the safety of high-frequency stimulation of the
subthalamic nucleus in rats. Journal of Neuroscience
Methods. 138(1-2): 207-16.
Kerner, T. E., Paulson, K. D., Hartov, A., Soho, S. K.,
Poplack, S. P., 2002. Electrical impedance
spectroscopy of the breast: clinical imaging results in
26 subjects. IEEE Transactions on medical imaging.
21(6): 638-45.
Kirik, D., Rosenblad, C., Björklund, A., 1998.
Characterization of behavioral and neurodegenerative
changes following partial lesions of the nigrostriatal
dopamine system induced by intrastriatal
6-hydroxydopamine in the rat. Experimental
Neurology. 152(2): 259-77.
Lempka, S. F., Miocinovic, S., Johnson, M. D., Vitek, J.
L., McIntyre, C. C., 2009. In vivo impedance
spectroscopy of deep brain stimulation electrodes.
Journal of Neural Engineering. 6, 046001, 11pp.
Lempka, S. F., Johnson, M. D., Moffittt, M. A., Otto, K.
J., Kipke, D. R., McIntyre, C. C., 2011. Theoretical
analysis of intracortical microelectrode recordings.
Journal of Neural Engineering. 8(4):045006.
Macdonald, J. R., 1992. Impedance spectroscopy. Annals
of Biomedical Engineering. 20(3): 289-305.
Nowak, K., Mix, E., Gimsa, J., Strauss, U.,
Sriperumbudur, K. K., Benecke, R., Gimsa, U., 2011.
Optimizing a rodent model of Parkinson’s disease for
exploring the effects and mechanisms of deep brain
stimulation. Parkinson’s Disease. 2011: 414682.
Wintermantel, E., Ha, S-W., 2002. Medizintechnik mit
biokompatiblen Werkstoffen und Verfahren. Springer,
Berlin, 3
rd
edition: 134-5.
Paxinos, G., Watson, C., The rat brain in stereotaxic
coordinates. Academic Press, San Diego, 1998.
BIOSIGNALS 2012 - International Conference on Bio-inspired Systems and Signal Processing
130