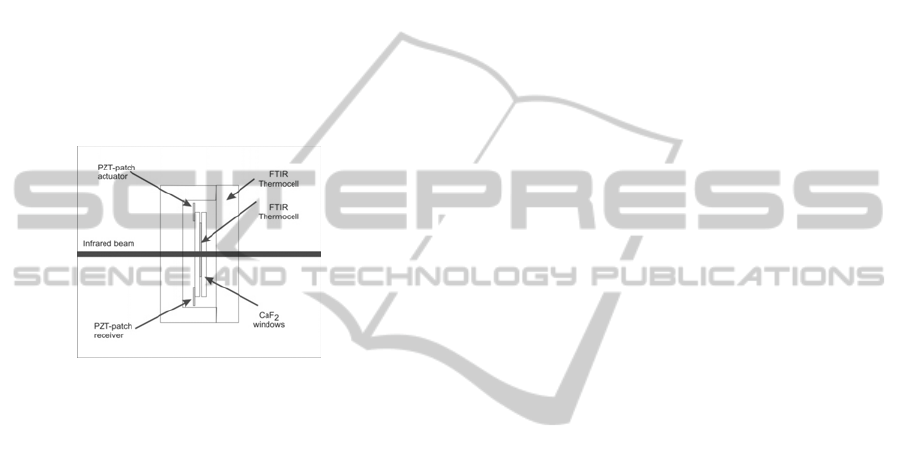
(PZT) transducers (with wrapped-around electrode,
provided by Meggit/InSensor®, Denmark) were
attached by epoxy resin adhesive, and the nickel
electrodes were wired to shielded contacts. The
liquid sample was loaded into the 5 x 5 mm opening
of the polytetrafluorethen spacer. The thickness of
the liquid layer was thus determined by the thickness
of the polytetrafluorethen gasket, d, which was in
the range of 50 micrometer. The CaF
2
plates were
closed and sealed by parafilm and vacuum grease to
prevent leakage of water. Since water loss,
especially induced by the heating of the sample,
would cause problems, in addition to these
measures, the stability of water concentration was
ensured by observing the intensity of the infrared
water peak.
Figure 1: Ultrasonic cell for in-situ spectroscopic
investigation of ultrasonic actuation. Scheme of operation
mode, vibrations in the CaF
2
sample assembly in the
transmission cell are generated by a PZT actuator and the
response is monitored by the PZT receiver.
The CaF
2
plate assembly was mounted in a
commercial SPECAC® temperature cell. In order to
avoid the transfer of essential amounts of ultrasonic
energy into the temperature cell, the ultrasonically
active plates were firmly embedded within heat-
resistant foam that isolated the plates due to the big
mismatch of acoustic impedances. Placing the foam
also reduced the thermal contact between the cell
and the probed region in the sample, resulting in an
offset between the registered temperature and the
actual sample temperature.
For the ultrasonic excitation, the following
approach was followed: a sinusoidal electrical signal
consisting of a continuous wave (cw) with a
frequency of about 50 kHz and an amplitude of 200
mV
pp
was generated by an Iwatsu SG-4511
pulse/function generator. The exact resonance
frequency was fine-tuned using the output signal
monitored at the oscilloscope. The electrical signal
from the generator was connected to the input of a
broadband amplifier (AR Worldwide 75A250). The
PZT transducer finally transformed the amplified
voltage signal into a mechanical oscillation that
released plate waves into the CaF
2
plates. Due to the
continuous operation mode, standing wave patterns
were generated by multiple reflections that depend
on the material, the frequency and the geometrical
boundary conditions of the sample holder. The
receiving PZT transducer was connected to a
LeCroy 9310 AM oscilloscope in order to monitor
the amplitude of the standing ultrasonic wave. For
connecting the electronic devices, shielded standard
BNC cables with an impedance of 50 Ohm were
used.
For driving the PZT transducer, a resonance
frequency was used that was fine-tuned by the
output signal. Whereas the main resonance
(thickness resonance) was in the range of 4 MHz, a
resonance around 50 kHz was selected.
According to a number of simplifications, the
sound intensity in the insulin solution can be
estimated. The final intensity estimated is in the
range of I = 180 mW/cm
2
given an average
displacement of ξ = 110 nm. Note that the threshold
of sound intensity in diagnostic medical applications
is also in the range of I = 100 mW/cm
2
, such as
mentioned above. On the other hand, the intensities
are too low to enable the forming of acoustic
cavitations. The present set-up thus meets the targets
of the intended study.
2.2 FTIR Spectroscopy
The unfolding and aggregation of insulin was
followed by FTIR-spectroscopy using a Bruker IFS-
66 spectrometer equipped with a liquid nitrogen-
cooled mercury cadmium telluride detector. 64
spectra with a resolution of 2 cm
-1
were co-added in
order to achieve a good signal to noise ratio. The
spectra were processed using the OPUS software of
the spectrometer, and the positions of the peaks were
determined using the peak seeking function of the
software. The temperature was controlled by both a
heating and cooling element, and it is measured by a
thermocouple that was attached at the thermocell.
The temperature was rising with a rate of
α
= 0.25
K/min. In this way, one obtains one spectrum for
every Kelvin that represents an average of the
spectroscopic signals in that temperature interval.
2.3 Preparation of Samples
Insulin from bovine pancreas [11070-73-8] was
obtained from Sigma Aldrich and was dissolved in
D
2
O [7789-20-0] obtained from Cambridge Isotope
Laboratories, Inc. (99.9%). The solvent D
2
O was
selected because the bending vibration of H
2
O
IN-SITU SPECTROSCOPIC INVESTIGATION OF UNFOLDING AND AGGREGATION OF INSULIN UNDER
ULTRASONIC EXCITATION - An Ultrasonic Actuator for FTIR-spectrometry on Biomatter
373