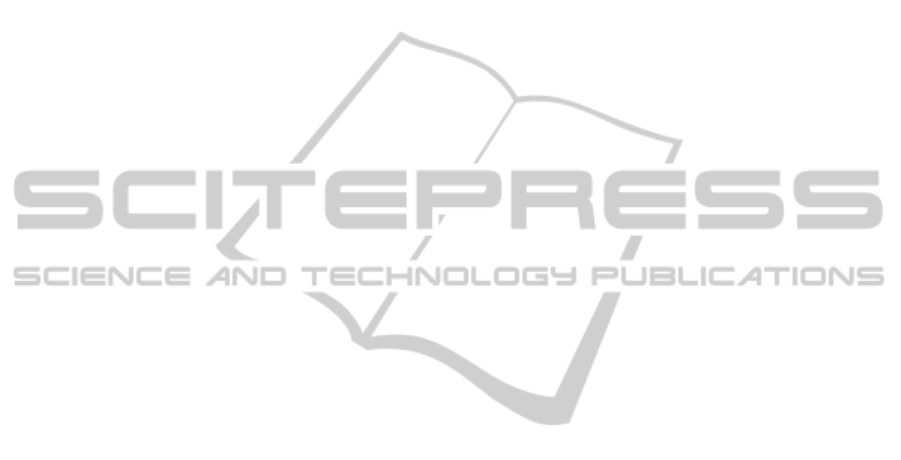
displacement of the trunk as attempting to reach the
target. Subject B presents increased anteriorization
of the trunk when compared to subject A. The
presence of a larger compensation at this level, in a
clinically less affected individual, could be related to
the difference in functional task completion.
Data analysis seems to suggest that the P1
position is advantageous for compensatory
movement detection at the shoulder level, being
however necessary to complement with information
provided by P5, in order to discriminate between
shoulder or trunk elevation. The information
provided by sensor locations P2 and P3 do not seem
to add relevant knowledge to that provided by sensor
position P1. The P4 position seems the most
appropriate for detecting the abduction component
of the limb; however, in relation with the superior-
inferior movement, this particular sensor position is
insufficient for determination of the corporal
segment where the elevation occurs
(shoulder/elbow/trunk), limiting its reliability for
compensatory movement identification in this
direction. Finally, sensor position P5 presents a good
sensitivity for anteriorization and rotation detection,
though lack of additional comparative data with
other locations at the trunk level.
5 CONCLUSIONS
Methods based on quantitative models can help
therapists and patients to effectively improve the
recovery process, by providing objective assessment
and monitoring, contributing to protocol validation
and information sharing. This preliminary study
focused on the determination of upper limb
associated compensatory movement through
accelerometry data and the influence of sensor
positioning.
ACKNOWLEDGEMENTS
The authors would like to thank the Foundation for
Science and Technology of Portugal for their
support of some of the PhD students involved in this
article (SFRH/BD/61396/2009 and
SFRH/BD/60929/2009). Additionally, the authors
would like to acknowledge the contribution of all
volunteers that took part of the testing procedures.
REFERENCES
Cirstea, M., Levin, M. 2007. Improvement of arm
movement patterns and end-point control depends on
type of feedback in stroke survivors.
Neurorehabilitation Neural Repair, 21, 398-411.
Cirstea, C., Ptito, A., Levin, M. 2006. Feedback and
cognition in arm motor skill reacquisition after stroke.
Stroke. 37, 1237-1242.
Fugl-Meyer, A. R., Jaasko, L., Leyman, I., Olsson, S.,
Steglind, S. 1975. The post-stroke hemiplegic patient.
I. A method for evaluation of physical performance.
Scand J Rehab Med 7, 13-31.
Geyh, S., Cieza, A., Schouten, J., Dickson, H., Frommelt,
P., Omar, Z., Kostanjsek, N., Ring, H., Stucki, G.
2004. ICF core sets for stroke. J Rehabil Med Suppl.
44, 135-141.
Levin, M. F., Desrosiers, J., Beauchemin, D., Bergeron,
N., Rochette, A. 2004. Development and Validation of
a Scale for Rating Motor Compensations Used for
Reaching Patients With Hemiparesis: The Reaching
Performance Scale. Physical Therapy. 84:1, 8-22.
Lucca, L. 2009. Virtual reality and motor rehabilitation of
the upper limb after stroke: a generation of progress? J
Rehabil Med. 41, 1003-1006.
Michaelsen, S.; Dannenbaum, R. and Levin, M. 2006.
Task-specific training with trunk restrain on arm
recovery in stroke – Randomized control trial. Stroke.
37, 186-192.
Michaelsen, S. A. Luta, A., Roby-Brami, A., Levin, M. F.
2001. Effect of trunk restraint on the recovery of
reaching movements in hemiparetic patients. Stroke.
32, 1875-1883.
Nudo, R. 2007. Post-infarct cortical plasticity and
behavioral recovery. Stroke. 38, 840-845.
Raine, S. 2009. The Bobath concept: developments and
current theoretical underpinning. In Raine, Meadows
and Lynch-Ellerington (eds). Bobath Concept –
Theory and clinical practice in neurological
rehabilitation. Wiley-Blackwell.
Reisman, D.S., Scholz, J. P. 2006. Workspace location
influences joint coordination during reaching in post-
stroke hemiparesis. Exp Brain Res. 170, 265-276.
Thrane, G., Emaus, N., Askim, T., Anke, A. 2011. Arm
use in patients with subacute stroke monitored by
accelerometry: association with motor impairment and
influence in self-dependence. J Rehabil Med. 43, 299-
304.
Vandenberghe, A., Levin, O., De Schutter, D., Swinnen, S.
Jonkers, I. 2010. Three-dimensional reaching tasks: effect
of reaching height and width on upper limb kinematics
and muscle activity. Gait & Posture. 32(4), 500-7.
Wagner, J. M., Lang, C. E., Sahrmann, S. A. Edwards, D.,
Dromerick, A. 2007. Sensorimotor impairments and
reaching performance in subjects with poststroke
hemiparesis during the first few months of recovery.
Physical Therapy. 87, 751-765.
World Health Organization. 2011. Stroke,
Cerebrovascular accident. Retrieved from www.who.
int/ topics/cerebrovascular_accident/en/
COMPENSATORY MOVEMENT DETECTION THROUGH INERTIAL SENSOR POSITIONING FOR
POST-STROKE REHABILITATION
301