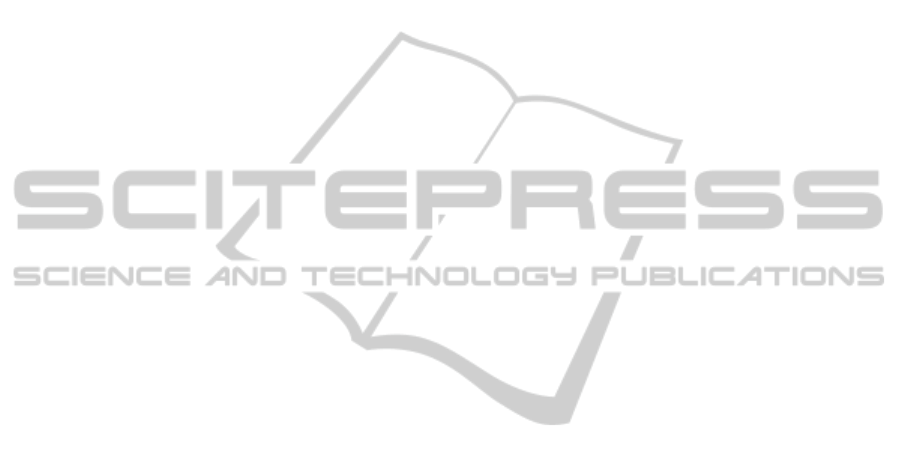
has yet to be confirmed. It is therefore too early to
describe this issue in further detail in this position
paper.
5 CONCLUSIONS
The ABO compatibility test is compulsory in
France. Studies led by the national hemovigilance
network show persistent attribution errors of blood
groups leading to transfusional accidents, with the
potential for death in each case (Afssaps, 2004). The
same observations have been made abroad
(Stainsby, 2005).The current procedure for
performing an ultimate pre-transfusional check is
not uniform. Although machines are sent to the
patient’s bedside for an automatic analysis of ABO
grouping, and technology has been developed to
attribute a code to the pouch of blood and to the
patient (Aandahl, 2007), these developments cannot
prevent human errors and transfusional accidents,
which can be lethal.
We have been working on a new system based
on biochips. We have shown through SPRi
techniques that these biochips are very selective and
can replace current hemagglutination test.
With our first tests with the SmarTTransfuseur,
we have shown that immunocapture is specific in
our conditions and that optical detection can be
carried out by absorption measurement. This
medical device could become a new uniform
procedure to carry out the ABO compatibility test.
Because tests will be carried out automatically,
human errors would be avoided and the additional
safety will involve only minor changes to current
practices.
We now need to repeat these tests in order to
cover all transfusional situations before clinical trials
in real-life situations.
The aim of this position paper is not only to
present the system we are developing, but also to
discuss the possibilities and techniques of ultimate
ABO compatibility tests outside France.
ACKNOWLEDGEMENTS
The authors would like to thank the French Blood
Transfusion Center, INSERM, the DGOS, the
CNRS, OSEO and the “innovative project
maturation” programme for their financial support.
REFERENCES
Aandahl, G. S., Knutsen, T. R., Nafstad, K., 2007.
Implementation of ISBT 128, a quality system, a
standardized bar code labeling of blood products
worldwide, electronic transfusion pathway: four years
of experience in Norway. Transfusion, 47(9), p.1674-
1678.
Afssaps, 2010. Rapport annuel hémovigilance. Available
at: http://www.afssaps.fr/.
Afssaps, 2004. Rapport annuel hémovigilance. Available
at: http://www.afssaps.fr/
Boireau, W. et al., 2009. Revisited BIA-MS combination:
entire «on-a-chip» processing leading to the proteins
identification at low femtomole to sub-femtomole
levels. Biosensors & Bioelectronics, 24(5), p.1121-
1127.
British Committee for Standards in Haematology, 2009.
Guideline on the Administration of Blood
Components. Available at: http://www.bcshguidelines.
com/documents/Admin_blood_components_bcsh_050
12010.pdf.
Dzik, W. H., 2007. New technology for transfusion safety.
British Journal of Haematology, 136(2), p.181-190.
Dzik, W. H., 2005. Technology for enhanced transfusion
safety. Hematology / the Education Program of the
American Society of Hematology. American Society
of Hematology. Education Program, p.476-482.
FDA, 2010. Fatalities Reported to FDA Following Blood
Collection and Transfusion. Available at: http://www.
fda.gov/downloads/BiologicsBloodVaccines/SafetyAv
ailability/ReportaProblem/TransfusionDonationFataliti
es/UCM254860.pdf.
Levy, G., 2008. [The pretransfusion bedside agglutination
test is not a «Gold Standard»]. Transfusion Clinique Et
Biologique: Journal De La Société Française De
Transfusion Sanguine, 15(5), p.318-321.
National Blood Collection and Utilization Survey, 2009.
The 2009 national blood collection and utilization
survey report. Available at: http://www.aabb.org/pro
grams/biovigilance/nbcus/Documents/09-nbcus-report.
Pazart L., Wacogne B., Pieralli C., Boireau W., Morel P.,
"Secure perfusion system", Patent WO 2011055031.
Pazart L., Wacogne B., Pieralli C., Boireau W., Morel P.,
"Device for taking a sample of a body fluid and
method for implementing same", Patent WO
2011055029.
SHOT, 2009. Annual Report. Available at: http://www.
shotuk.org/
Stainsby, D., 2005. ABO incompatible transfusions--
experience from the UK Serious Hazards of
Transfusion (SHOT) scheme Transfusions ABO
incompatible. Transfusion Clinique Et Biologique:
Journal De La Société Française De Transfusion
Sanguine, 12(5), p.385-388.
Stenberg, E., 1991. Quantitative determination of surface
concentration of protein with surface plasmon
resonance using radiolabeled proteins. Journal of
Colloid and Interface Science, 143(2), p.513-526.
BIODEVICES 2012 - International Conference on Biomedical Electronics and Devices
262