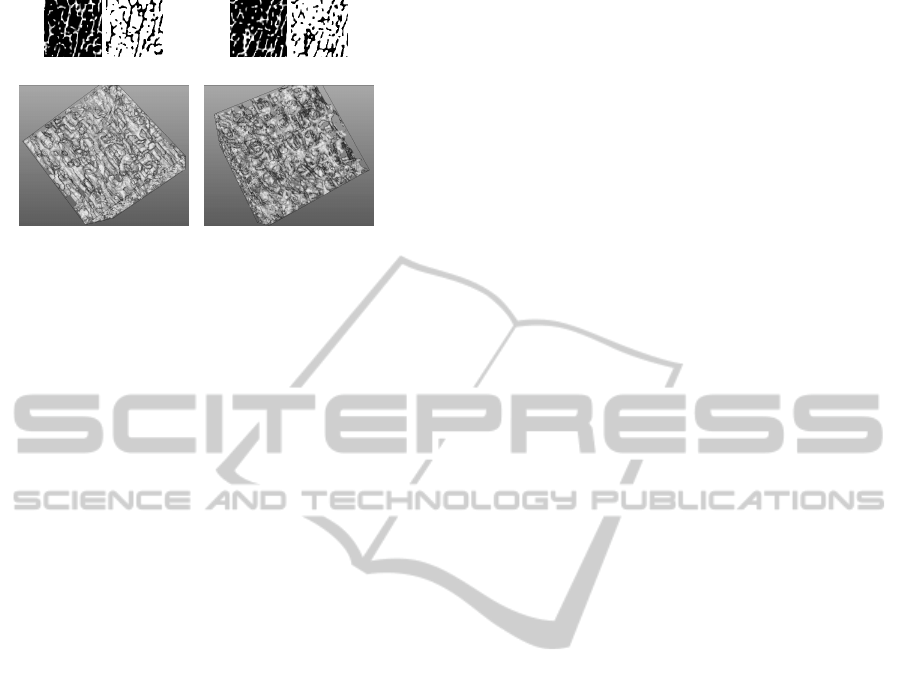
(a) Exemplars + binary masks.
(b) Generated volume. (c) Real volume.
Figure 8: Anisotropic synthesis with additional binary
masks. Surface rendering of trabecular bone architecture
obtained with the synthetic texture and the SRµCT image.
line. It can be noticed that for all the morphologic pa-
rameters the score for the virtual bone is spread in a
range lower than +/- one standard deviation from the
mean value obtained from the SRµCT set, thus prov-
ing its resemblance with the architecture of real bone.
For the topology parameter (Euler/mm3), the score is
slightly higher than the maximum value obtained with
the SRµCT set, but that remains satisfying. It means
that the virtual bone structure has a somewhat lower
connectivity than the reference set. It could represent
a high degree of osteoporosis.
In Figure 8, the surface of the virtual bone is com-
pared to the surface of the 3D SRµCT image from
which were taken the reference slices for the texture
synthesis. We retrieve visually the similarity demon-
strated by the bone parameters.
5 CONCLUSIONS
Our method allows to create realistic organic tis-
sue from small samples acquired by a digital micro-
scope or other image acquisition devices such as Syn-
chrotron Radiation Computed Micro-Tomography.
We demonstrated quantitatively the accuracy of the
synthetic texture from both statistical and morpho-
logical points of view. Latter tests showed that our
synthetic objects like the zebra-dot 3D texture can be
useful for simulation especially in the framework of
Brownian simulation. In future work, we will use our
method to enhance surface models. We will aim at
coupling texture synthesis with minimal skeleton rep-
resentations such as the m-reps developed by Pizer et.
al (Pizer et al., 2003) and reproducing the interior of
the organs at multi-scale levels using 2D samples of
tissue.
REFERENCES
Heeger, D. J. and Bergen, J. R. (1995). Pyramid-based tex-
ture analysis/synthesis. In Proceedings of the 22nd
annual conference on Computer graphics and inter-
active techniques, SIGGRAPH ’95, pages 229–238,
New York, NY, USA. ACM.
Hipp, J. and Simmons, C. (1997). Method-based dif-
ferences in the automated analysis of the three-
dimensional morphology of trabecular bone. Journal
of Bone and Mineral Research, 12.
Kopf, J., Fu, C.-W., Cohen-Or, D., Deussen, O., Lischinski,
D., and Wong, T.-T. (2007). Solid texture synthesis
from 2d exemplars. ACM Transactions on Graphics
(Proceedings of SIGGRAPH 2007), 26(3):2:1–2:9.
Kwatra, V., Essa, I., Bobick, A., and Kwatra, N. (2005).
Texture optimization for example-based synthesis.
ACM Transactions on Graphics, SIGGRAPH 2005.
Lefebvre, S. and Hoppe, H. (2006). Appearance-space tex-
ture synthesis. ACM Trans. Graph., 25:541–548.
Odgaard, A. and Gundersen, H. (1993). Quantification of
connectivity in cancellous bone, with special empha-
sis on 3d reconstructions. Bone, 14:173–182.
Pizer, S. M., Fletcher, P. T., Joshi, S., Thall, A., Chen,
J. Z., Fridman, Y., Fritsch, D. S., Gash, A. G., Glotzer,
J. M., Jiroutek, M. R., Lu, C., Muller, K. E., Tracton,
G., Yushkevich, P., and Chaney, E. L. (2003). De-
formable m-reps for 3d medical image segmentation.
Int. J. Comput. Vision, 55:85–106.
Revol-Muller, C., Benoit-Cattin, H., Carillon, Y., Odet, C.,
Briguet, A., and Peyrin, F. (2002). Bone mri seg-
mentation assessment based on synchrotron radiation
computed microtomography. IEEE Trans Nucl Sci,
49(1):220–224.
Rolland, J. P., Vo, V., Bloss, B., and Abbey, C. K. (2000).
Fast algorithms for histogram matching: application
to texture synthesis. Journal of Electronic Imaging,
9:39–45.
3D TEXTURE SYNTHESIS FOR MODELING REALISTIC ORGANIC TISSUES
65