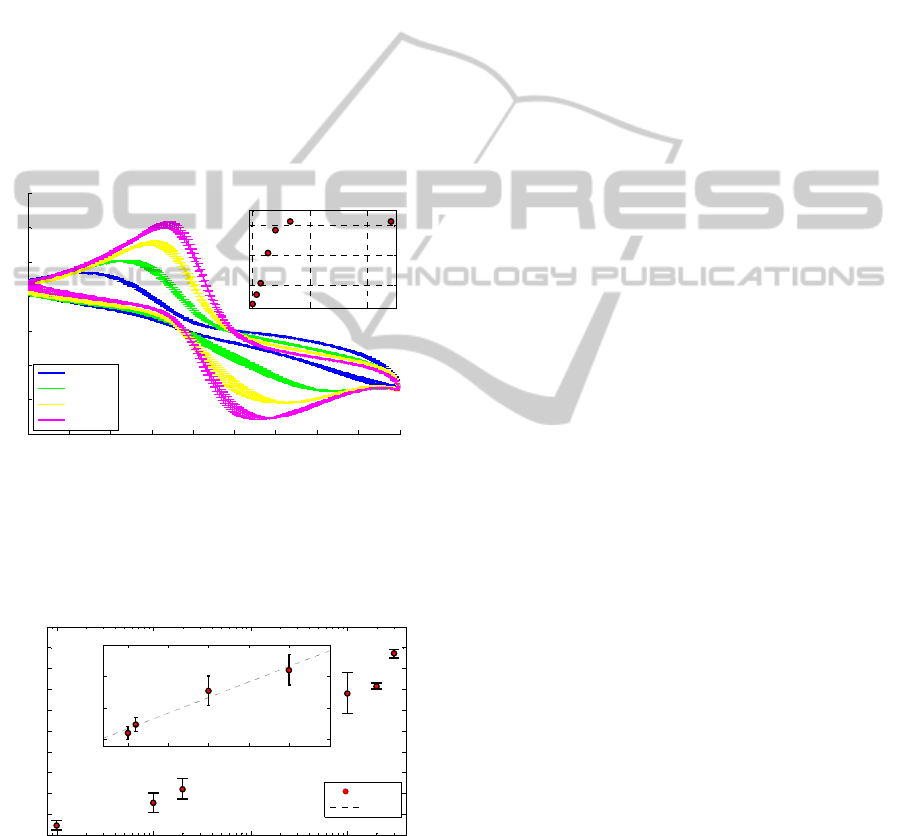
with EIS measurements in concentration range
between 1 mg/mL up to 25 mg/mL. An equivalent
electrical model based on a weighted contribution
approach has been developed in order to obtain a
numeric parameter that is related to the electrode
coverage. Both this methodology and CVM
measurements have identified in 10 mg/mL the
enzyme concentration that leads to adequate
electrodes coverage. The sensors lactic acid
detection performance have been evaluated using
CVM measurements: the upper saturation levels is
reached at about 300 mM, and the sensitivity is
about 6.24 µA mM
-1
cm
-2
.
This work is part of a University of Padova
research project for the development of an
innovative cell-based Lab-On-Chip for clinical and
food-processing massive screening applications.
Figure 6: CVM response of 2 mm diameter gold sensors
with different LA concentration in measurement solution.
Box: CVM reduction peaks currents as a function of LA
concentrations. The response saturates for LA
concentrations above 300 mM.
Figure 7: CVM reduction peaks currents as a function of
LA concentrations for round gold electrodes (1 mm
diameter). The tested LA concentrations vary from 1 μm
up to 300 mM. Box: magnification in milli-molar range.
Regression line: i
p,red
= 4.9 10
-2
C
LA
+ 1.1 10
-6
.
ACKNOWLEDGEMENTS
This project is partially supported by the University
of Padova project “Design and validation of a
biosensor to monitor myogenic cell growth in vitro”
(Department of Human Anatomy and Physiology,
Department of Information Engineering). This
project is partially supported by University of
Padova project MISCHA (Microfluidic laboratory
for Scientific and teCHnological Applications).
REFERENCES
Boujtita, M.; Chapleau, M. and Mum, N. E. (1996)
Enzymatic Electrode for the Determination of L-
Lactate, Electroanalysis, 8 (5), 485-488.
Castillo, J. and Gáspár, S. (2004). Biosensors for life
quality: Design, development and applications.
Sensors and Actuators B, 102, 179-194.
Gamero, M. and Pariente, F. (2010). Nanostructured rough
gold electrodes for the development of lactate oxidase
– based biosensor. Biosensors and Bioelectronics, 25,
2038-2044.
Huang, X.; Nguyen D.; Greve, D. W. and Domach M. M.
(2004). Simulation of Microelectrode Impedance
changes Due to Cell Growth, IEEE Sensors Journal, 5
(4), 576-583.
Jena, B. K. and Raj, C. R. (2006) Electrochemical
Biosensor Based on Integrated Assembly of
Dehydrogenase Enzymes and Gold Nanoparticles,
Anal. Chem., 78, 6332-6339.
Lee, H. C.; Wu, W. Y.; Lin, J. L.; Chin, Y. L.; Lee, K. Y.
and Sun, T. P. (2008) Evolution of the TiO2
membrane on ITO PET substrate applied to a lactate
biosensor using a potentiometric differential readout,
circuit Sensors, 2008 IEEE, 898-901.
Onaral, B.; Sun H. H. and Schwan, H. P. (1984). Electrical
Properties of Bioelectrodes, IEEE Transactions on
Biomedical Engineering, 12 (BME-31), 827-832.
Palmisano, F.; Quinto M; Rizzi R. and Zambonin (2001)
Flow injection analysis of L-lactate in milk and
yoghurt by on-line microdialysis and amperometric
detection at a disposable biosensor, Analyst, 126, 866-
870.
Parra, A. and Casero, E. (2006). Design and characteri-
zation of a lactate biosensor based on immobilized
lactate oxidase onto gold surfaces. Analytica Chimica
Acta, 555, 308-315.
Sung, W. J. and Bae, Y. H. (2006) Glucose oxidase,
lactate oxidase, and galactose oxidase enzyme
electrode based on polypyrrole with polyanion/PEG/
enzyme conjugate dopant, Sensors and Actuators B,
114, 164-169.
-0.2 -0.1 0 0.1 0.2 0.3 0.4 0.5 0.6 0.
-6
-4
-2
0
2
4
6
8
x 10
-6
Voltage [V]
Current [A]
w/o LA
0.07 mM
0.13 mM
1.2 mM
0 0.5 1
4
5
6
x 10
-6
C
LA
[M]
i
p,red
[A]
0 0.5 1 1.5 2 2.5
-2
0
2
x 10
-7
residuals
10
-4
10
-3
10
-2
10
-1
1.15
1.2
1.25
1.3
1.35
1.4
1.45
1.5
1.55
x 10
-6
C
LA
[M]
i
p,red
[A]
0 0.5 1 1.5 2 2.5
1.1
1.15
1.2
1.25
x 10
-6
C
LA
[mM]
i
p,red
[A]
data
linear
LOW-COST ENZYME-BASED BIOSENSOR FOR LACTIC ACID AMPEROMETRIC DETECTION - Electrical
Modeling and Validation for Clinical and Food Processing Applications
383