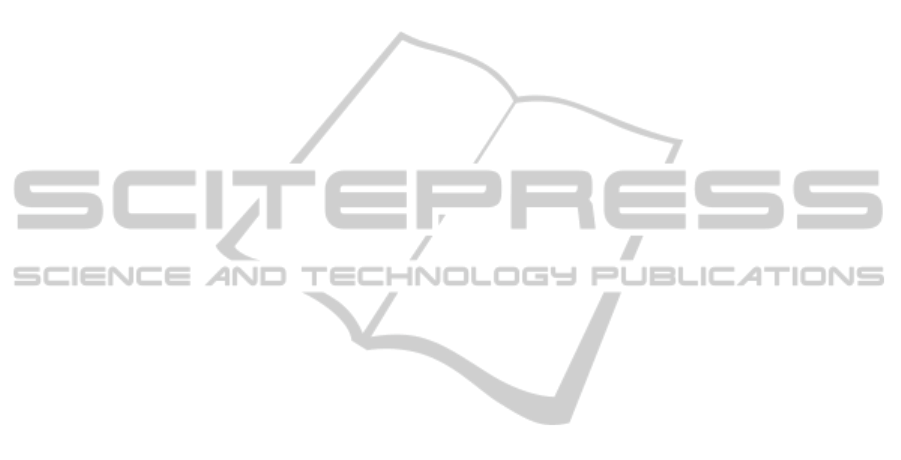
Acknowledgements
This investigation was supported by FEDER funds trough the COMPETE Program,
and by national funds from Portuguese Foundation for Science and Technology, un-
der the project COMPETE: FCOMP-01-0124-FEDER-009525
(PTDC/CVT/101999/2008) and COMPETE: FCOMP-01-0124-FEDER-009497
(PTDC/CVT/099022/2008).
References
1. A. Silva, S. Campos, J. Monteiro, C. Venancio, B. Costa, P. Guedes de Pinho, and L. An-
tunes, “Performance of anesthetic depth indexes in rabbits under propofol anesthesia: pre-
diction probabilities and concentration-effect relations,” Anesthesiology, vol. 115, no. 2,
2011, pp. 303-314.
2. A. Silva, D.A. Ferreira, C. Venancio, A. P. Souza, and L. M. Antunes, “Performance of
electroencephalogram-derived parameters in prediction of depth of anaesthesia in a rabbit
model,” Br J Anaesth, vol. 106, no. 4, 2011, pp. 540-547.
3. M. Wright, “Pharmacologic effects of ketamine and its use in veterinary medicine,” J Am
Vet Med Assoc, vol. 180, no. 12, 1982, pp. 1462-1471.
4. G. J. Benson, and J. C. Thurmon, “Intravenous anesthesia,” Vet Clin North Am Equine
Pract, vol. 6, no. 3, 1990, pp. 513-528.
5. J. Thurmon, W. Tranquilli, G. Benson, W. Lumb, and E. Jones, Lumb and Jones' Veteri-
nary Anesthesia, 1996.
6. C. Valtolina, J. H. Robben, J. Uilenreef, J. C. Murrell, J. Aspegren, B. C. McKusick, and L.
J. Hellebrekers, “Clinical evaluation of the efficacy and safety of a constant rate infusion of
dexmedetomidine for postoperative pain management in dogs,” Vet Anaesth Analg, vol. 36,
no. 4, 2009, pp. 369-383.
7. G. Y. Lin, J. H. Robben, J. C. Murrell, J. Aspegren, B. C. McKusick, and L. J. Hellebrek-
ers, “Dexmedetomidine constant rate infusion for 24 hours during and after propofol or
isoflurane anaesthesia in dogs,” Vet Anaesth Analg, vol. 35, no. 2, 2008, pp. 141-153.
8. J. Bruhn, P. S. Myles, R. Sneyd, and M. M. Struys, “Depth of anaesthesia monitoring:
what's available, what's validated and what's next?,” Br J Anaesth, vol. 97, no. 1, 2006, pp.
85-94.
9. P. A. March, and W. W. Muir, “Bispectral analysis of the electroencephalogram: a review
of its development and use in anesthesia,” Vet Anaesth Analg, vol. 32, no. 5, 2005, pp. 241-
255.
10. M. Revuelta, P. Paniagua, J. M. Campos, J. A. Fernandez, A. Martinez, M. Jospin, and H.
Litvan, “Validation of the index of consciousness during sevoflurane and remifentanil
anaesthesia: a comparison with the bispectral index and the cerebral state index,” Br J
Anaesth, vol. 101, no. 5, 2008, pp. 653-658.
11. X. Li, S. Cui, and L. J. Voss, “Using permutation entropy to measure the electroencephalo-
graphic effects of sevoflurane,” Anesthesiology, vol. 109, no. 3, 2008, pp. 448-456.
12. A. Silva, H. Cardoso-Cruz, F. Silva, V. Galhardo, and L. Antunes, “Comparison of anes-
thetic depth indexes based on thalamocortical local field potentials in rats,” Anesthesiology,
vol. 112, no. 2, 2010, pp. 355-363.
13. Morpheus-Medical, “IoC-View version 2.1 user manual,” Morpheus Medical 2008
14. C. Bandt, and B. Pompe, “Permutation entropy: a natural complexity measure for time
series,” Phys Rev Lett, vol. 88, no. 17, 2002, pp. 174102.
50