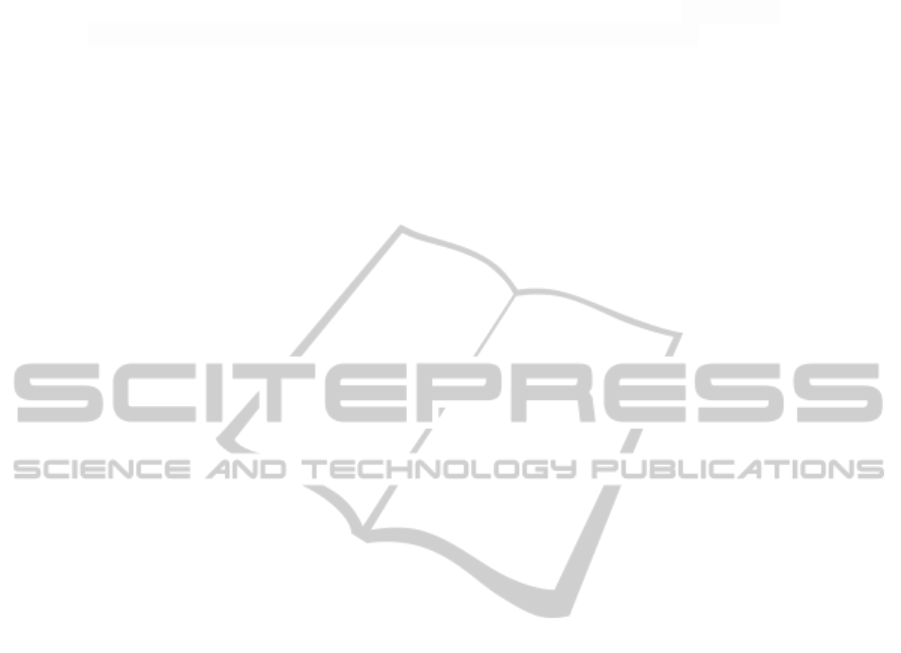
that led to the concept of MAC (Minimum Alveolar Concentration) and provides the
best available method to monitor continuous brain concentration, nowadays.
Pharmacodynamic properties of propofol depend on its therapeutic plasma
concentrations. The knowledge of pharmacokinetic models allows a more accurately
prediction of the optimal dosage [9]. The required plasma concentration varies with
the desired pharmacological effect (sedation, induction or maintenance of
anaesthesia), the simultaneous use of other drugs (opioids, muscle relaxants), the type
of operation and the patient’s sensitivity to the drug (age, weight and pre-existing
diseases) [9]. So, the use of propofol demands a continuous titration of the drug
infusion rate to the desired pharmacological end point, however there is an absence of
a clinically useful method for measuring blood propofol concentrations.
In this study, an analysis over real propofol concentrations together with predicted
concentrations was performed using a rabbit model.
2 Methodology
2.1 Animals
All procedures were carried out under personal and project licenses approved by the
national regulatory office (Direcção Geral de Veterinária – DGV). Three healthy male
New Zealand White rabbits, approximately 2 months old, were used.
2.2 Anaesthetic Protocol
Anaesthesia was induced with a dose of 20 mg.kg
-1
propofol (Propofol Lipuro®, B.
Braun Melsungen, Germany) intravenously, using a syringe pump (Asena GH, Alaris
Medical Systems) controlled by the Rugloop II software (developed by Tom DeSmet
(Demed Engineering, Gent, Belgium)) at an infusion rate of 200 ml.h
-1
. Following
blind orotracheal intubation with a cuffed endotracheal tube with 2.5 mm in internal
diameter, propofol started to be administered according to an infusion scheme in
which three infusion rates were used in every animal: each infusion (70, 100 and 130
mg.kg
-1
.h
-1
) was maintained during thirty minutes. The order of the administration
rates chosen for each animal was random.
Monitoring of anaesthesia included cardio-respiratory parameters (heart rate (HR),
mean arterial blood pressure (MABP), arterial blood oxygen (SpO
2
) and respiratory
rate (RR)), temperature (T), clinical evaluation of depth of anaesthesia (DoA) and the
Index of Consciousness (IoC).
Anaesthetic monitoring included cardio-respiratory monitoring provided by a
Datex S/5 Anaesthetic station (Datex Ohmeda, Helsinki, Finland) which included:
pulse-oxymetry and pulse rate monitored with the probe placed in the ear, invasive
mean arterial blood pressure (MBAP), inspired and end-tidal concentrations of
oxygen and carbon dioxide. The animals were under mechanical ventilation with
100% oxygen, with ventilation parameters set to maintain the end-tidal CO
2
(ETCO
2
)
between 35 and 45 mmHg. At the end of the infusion scheme fresh gas flow rate was
19