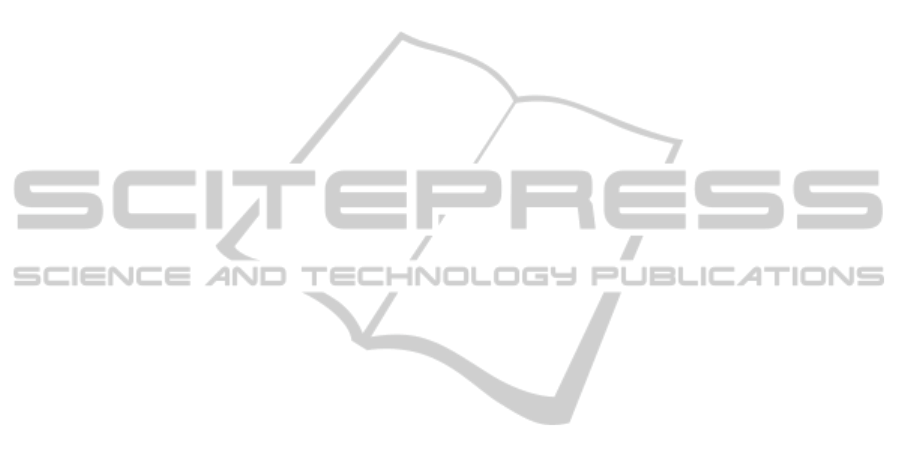
References
1. Tortora, G. J, Grabowski, S. R., (2001). Introduction of Human Body – The essentials of
anatomy and Physiology. John Wiley & Sons, INC, 5th Edition.
2. Paoletti, M., Marchesi, C., (2006). Discovering dangerous patterns in long-term ambulatory
ECG recordings using a fast QRS detection algorithm and explorative data analysis. Com-
puter Methods and Programs in Biomedicine, 82:20-30.
3. Macfarlane, P., Colaco, R., Stevens, K., Reay, P., Beckett, C., and Aitchison, T., (2003).
Precordial electrode placement in women. Netherlands Heart Journal, 11(3):118.
4. Soliman, E. Z., (2008). A simple measure to control for variations in chest electrodes
placement in serial electrocardiogram recording. Journal of Electrocardiology, 41:378-379.
5. García-Niebla, J., Llontop-García, P., Valle-Racero, J. I., Serra-Autonell, G., Batchvarov,
V. N., (2009). Technical mistakes during the acquisition of the Electrocardiogram. Journal
compilation, Willey Periodicals, Inc, 14(4).
7. Muhlsteff, J., Such, O., Schmidt, R., Reiter, H., Lauter, J., Thijs, J., Musch, G., Harris, M.,
(2004). Wearable approach for continuous ECG – and Activity Patient-Monitoring. Pro-
ceedings of the 26th Annual Internat.Conf. of IEEE EMBS, San Francisco, CA, USA:1-5.
8. Mahmoud, S. S., Fang, Q., Davidovic, D. M., Cosic, I., (2006). A Time-Frequency ap-
proach for the analysis of Normal and Arrythmia Cardiac Signals. Proceeding of the 26th
IEEE EMBS Annual International Conference, New Work City, USA.
9. PLUX, ecgPLUX, PLUX Wireless Biosignals. Available from: www.plux.info [Accessed
August 8, 2011].
10. PLUX, bioPLUX Research, PLUX Wireless Biosignals. Available from: www.plux.info
[Accessed Aug 8, 2011].
11. Rautaharju, P., Park, L., Rautaharju, F., and Crow, R., (1998). A standardized procedure
for locating and documenting ecg chest electrode positions: consideration of the effect of
breast tissue on ecg amplitudes in women. Journal of electrocardiology, 31(1):17–29.
12. Horton, J., Sherber, H., and Lakatta, E., (1977). Distance correction for precordial electro-
cardiographic voltage in estimating left ventricular mass. Circulation, 55(3):509.
13. Mohammed, M. (2001). Determination of left ventricular mass by echocardiography in
normal Arab people. morbidity and mortality, 4:6.
14. Peralta-Huertas, J., Livingstone, K., Banach, A., Klentrou, P., and O’Leary, D., (2008).
Differences in left ventricular mass between overweight and normal-weight preadolescent
children. Applied Physiology, Nutrition, and Metabolism, 55.
15. Pan, J, Tompkins, W. J., (1985). A real-time QRS detection algorithm. IEEE Transactions
on Biomedical Engineering, 3:230-236.
88