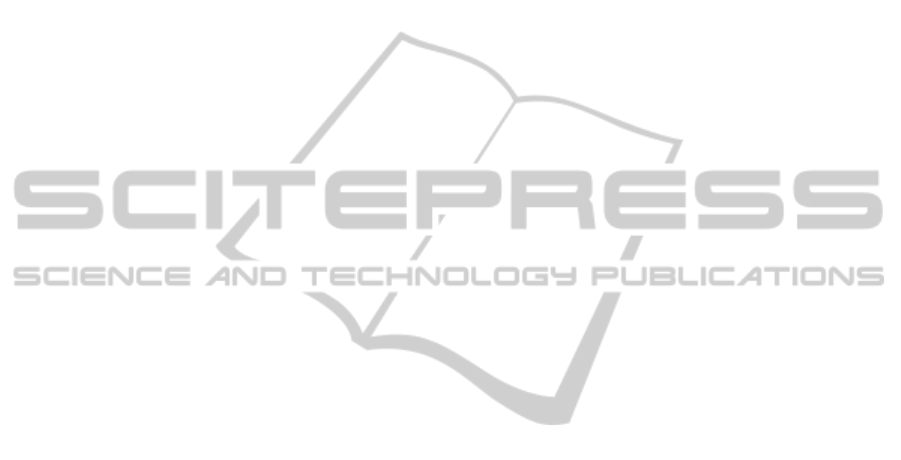
References
1. Urbain, B., Gustin, P., Charlier, G., Coignoul, F., Lambotte, J. L., Grignon, G., Foliguet,
B., Vidic, B., Beerens, D., Prouvost, J. F. and Ansay, M.: A morphometric and functional
study of the toxicity of atmospheric ammonia in the extrathoracic airways in pigs. Vet. Res.
Commun. (1996, a) 20, 381–399.
2. Urbain, B., Provoust, J. F., Beerens, D., Michel, O., Nicks, B., Ansay, M., Gustin, P.:
Chronic exposure of pigs to airborne dust and endotoxins in an environmental chamber:
technical note. Vet. Res. Commun. (1996, b) 27, 569–578.
3. Ferrari S., Silva M., Guarino M., Berckmans D.: Analysis OF Cough Sounds for Diagnosis
of Respiratory infections in Intensive Pig Farming. Transaction of the Asabe. (2008a)
51(3), 1051-1055(6).
4. Van Hirtum, A., Berckmans, D.: Considering the influence of artificial environmental noise
to study cough time-frequency features. Journal of Sound and Vibration. (2003a) 266 (3),
667-675.
5. Van Hirtum, A., Berckmans, D.: Fuzzy approach for improved recognition of citric acid
induced piglet coughing from continuous registration. Journal of Sound and Vibration.
(2003b) 266 (3), 677-686.
6. Guarino M., Costa A., Van Hirtum A., Jans P., Ghesquiere K., Aerts J. M., Berckmans D.:
Field tests of an algorithm to predict infected pig coughing. Annals of Animal Science.
Suppl. (2004) 1, 61-65.
7. Silva M., Ferrari S., Costa A., Aerts J. M., Guarino M., Berckmans D.: Cough localization
for the detection of respiratory diseases in pig houses. Computers and Electronics in Agri-
culture. (2008) 64, 286–292.
8. Exadaktylos V., Silva M., Ferrari S., Guarino M., Aerts JM, Berckmans D.: Time–series
analysis for online recognition and localization of sick pig (Sus scrofa) cough sounds.
J.Acoust. Soc. Am.(2009) 124 (6), 3803-3809.
9. Svensson C., Liberg P., Hultgren J.: Evaluating the efficacy of serum haptoglobin concen-
tration as an indicator of respiratory-tract disease in dairy calves. The Veterinary Journal.
(2007) 174, 288-294.
10. Kelley K.W.: Stress and immune function: a bibliographic review. Ann. Rech. Vet. (1980)
11:445-478.
Kelley, K.
39