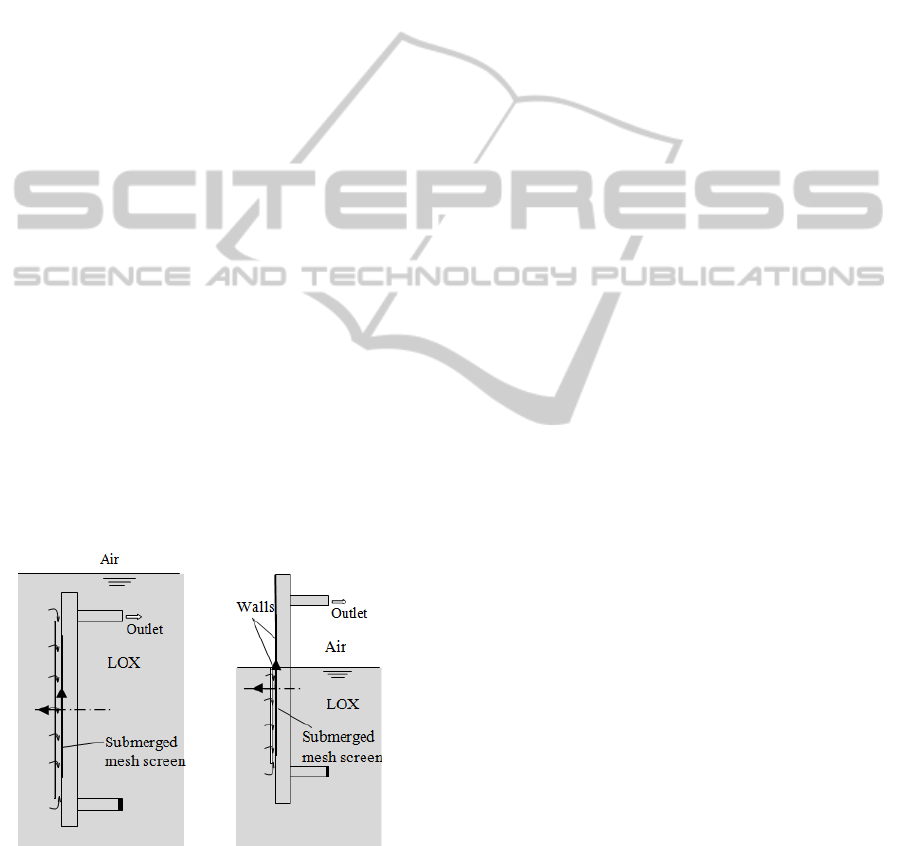
drop between the vapor and the LOX does not
exceed the bubble point.
The pressure-based solver was used, with a
steady implicit formulation. The three-dimensional
simulations used the Green-Gauss cell based
gradient and the standard k-epsilon model for the
turbulent flow with the following constants: The
coefficient of first order turbulent dissipation rate in
the dissipation rate equation, C
1
ε
= 1.44; the
coefficient of second order turbulent dissipation rate
in the dissipation rate equation C
2
ε
= 1.92; the
turbulent viscosity coefficient C
μ
= 0.09; the
turbulent Prandtl number for turbulent kinetic
energy,
σ
k
= 1.0; and the turbulent Prandtl number
for dissipation rate of turbulent kinetic energy,
σ
ε
=
1.3.
The operating conditions were set to a system
pressure = 1620269 Pa, T= 89 K, and gravitational
acceleration vectors were set at g
x
= g
z
= 0.0 m/s
2
,
and g
y
= -9.81 m/s
2
for the horizontally-oriented
completely submersion cases, while g
y
= g
z
= 0.0
m/s
2
, and g
x
= -9.81 m/s
2
for vertically-oriented
completely and partially submerged cases.
The ‘pressure-outlet’ type was selected as the
boundary condition at the flow outlet, and set to a
gauge pressure of 0 Pa with a backflow modified
turbulent viscosity of 0.001 m
2
/s. The ‘pressure-inlet’
type boundary condition was selected at the flow
inlet surface with corresponding values of total
gauge pressure and modified turbulent viscosity of
0.001 m
2
/s to obtain a mass flow rates within the
range of 0.0455 kg/s (0.1 lbs/s) ~ 0.1818 kg/s (0.4
lbs/s). It
should be noted that all the gauge pressures
are relative to the operating pressure.
Figure 3: Vertically submerged LAD channel assembly.
4 SIMULATION RESULTS
Twenty four cases of vertically-oriented submerged
LAD channel, including six complete submerged,
seven 2/3 submerged screen length, five 1/2
submerged screen length, and six 1/3 submerged
screen length cases, were simulated. Two cases of
horizontally-oriented submerged LAD channel were
simulated for comparison with the corresponding
vertically-oriented completely submerged cases. The
simulation results show that for the completely
submerged cases, the mass flow rate passing through
the LAD channel is not affected by the orientation of
the channel. In fact, the total static pressure drop
between the inlets and outlet,
Δ
p
i-o
, and submerged
portion of the screen determines the flow rate. For
example, at the cases of completely submerged
channel, the value of
Δ
p
i-o
is proportional to the
mass flow rate and independent of channel
orientation However, as more of the screen becomes
exposed in the vertically-oriented submerged cases,
a larger
Δ
p
i-o
is needed to achieve the same mass
flow rate. As is well known, the flow pattern in the
channel directly reflects the effects of gravity on the
channel performance. In order to ascertain the
effects of the channel orientation or gravity on the
LAD channel performance, the velocity vector fields
are visualized and compared to reveal the flow
pattern characteristics.
4.1 Velocity Vector Field Visualization
For the case of vertically-oriented, completely
submerged channel, the hydrostatic pressures in both
entrance gap and LAD flow channel are the same;
therefore, gravity has no effect on the flow in the
channel. The flow pattern in the channel is no
different between the completely submerged cases
horizontally and vertically oriented channels. For
example, the velocity vectors near the outlet tube for
both cases are almost identical, as shown in Figure 4.
The velocity vectors for completely submerged
channels along the channel length at the sections of
axial distance of x = -0.16 m, x = 0 m, and x = 0.16
m, are also identical for horizontally and vertically
oriented channels, respectively, as shown in Figures
5a, 45b, and 5c, in which the left ones are for
horizontal orientation while the right ones for
vertical orientation. However, gravity affects the
flow pattern in the flow channel when a portion of
the screen is exposed. At the same
Δ
p
i-o
, the amount
of exposed screen length is proportional to the
hydrostatic pressure drop between the entrance gap
and the LAD flow channel; consequently, when the
screen exposed portion increases, the resistance of
the flow passing through the flow channel becomes
larger. As a result, the flow in the flow channel by
VELOCITY VECTOR FIELD VISUALIZATION OF FLOW IN LIQUID ACQUISITION DEVICE CHANNEL
745