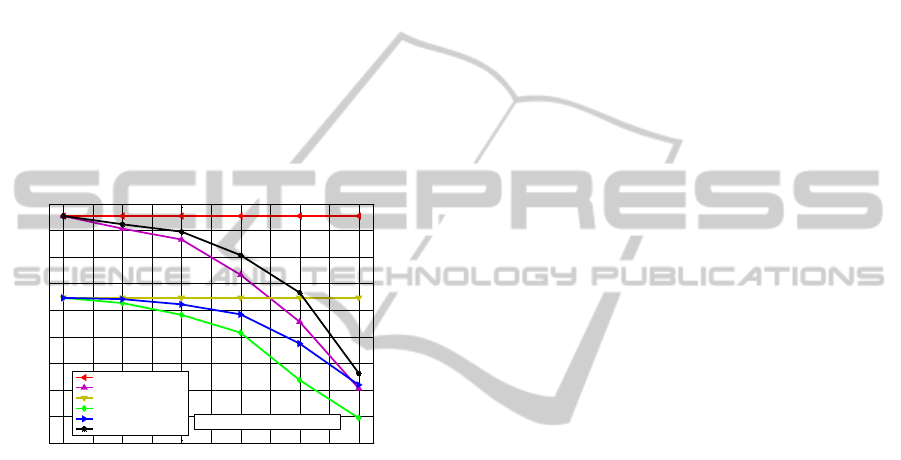
wavelength and spacing also measured to verify
colorless operation and applicability of the FSR
periodicity of AWG as shown in Figure 4 (c) and (d).
The measured optical carrier range from 1532.5 nm
to 1572.5 nm (equivalent to almost entire C band),
the maximum achievable data rate was maintained
almost 20 Gb/s at the RSOA and preamplifier input
optical power of -7 dBm and -3 dBm, respectively.
This maximum achievable data rate slightly
decreased at about 1570 nm because gain
characteristic of EDFA in preamplifier has poor gain
characteristic at this wavelength. For this reason, the
optical receiver sensitivity also became worse and
led to the performance penalty. The I/Q wavelength
difference has no influence on the maximum
achievable data rate as shown in Figure 4 (d). These
data rates are similar to the average of low and high
wavelength channel performance.
Figure 5: Maximum achievable data rate with various
input optical power deviation of the preamplifier power
between I /Q channels.
The signal performance was also evaluated as a
function of the input preamplifier power deviation
between I/Q channels as represented in Figure 5. In
this figure, “high” channel means either I or Q
channel which had higher input optical power at the
preamplifier, and vice versa. The measurement
curves labeled “measured” represents the signal
performance after combining I/Q channels (in other
words, “high” and “low” channels) with a given
input preamplifier power deviation. It was verified
that the signal performance was degraded as the
power deviation was increased. As a whole, the
performance degradation followed the “low”
channel’s characteristic. However, this performance
degradation was almost negligible when the
deviation was less than 12 dB, which led to 1-Gb/s
performance penalty compared to the case of no
power deviation. This 12-dB deviation margin was
high enough to provide consistent data rate
transmission in the proposed scheme because both
I/Q channels indeed shared the same link and
consequently, there was no significant input
preamplifier power difference between I/Q channels.
4 CONCLUSIONS
We successfully demonstrated the novel
transmission scheme to provide 20-Gb/s optical
OFDM signal through the 20-km SMF based on the
1-GHz bandwidth-limited RSOAs for source free
and colorless ONU. The I and Q channels were
separated in wavelength domain and independently
transmitted at baseband without Hermitian
Symmetry. The CW optical sources for upstream
signal were generated at OLT pass through bi-
directional link for source free ONU. We reported a
proof-of-concept experiment to show the
applicability of FSR periodicity of AWG. The
OFDM symbol with adaptive bit and power loading
was applied to overcome the bandwidth limitation of
the RSOA. This solution is promising for low-cost
20 Gb/s upstream transceiver for colorless ONU of
>10G-PON.
REFERENCES
Lee, C.-H., Sorin, W. V., Kim, B.-Y., 2006, Fiber to the
home using a PON infrastructure, Journal of
Lightwave Technology, 24(12), pp. 4568-4583.
Papagiannakis, I., Omella, M., Klonidis, D., Birbas, A., N.,
Kikidis, J., Tomkos, I., Prat, J., 2008, Investigation of
10-Gb/s RSOA-based upstream transmission in
WDM-PONs utilizing optical filtering and electronic
equalization, IEEE Photonics Technology Letters,
20(24), pp. 2168-2170.
Cho, K. Y., Choi, B. S., Takushima, Y., Chung, Y. C.,
2011, 25.78-Gb/s operation of RSOA for next-
generation optical access networks, IEEE Photonics
Technology Letters, 23(8), pp. 495-497.
Valicourt, G. de, Make, D., Fortin, C., Enard, A., Van Dijk,
F., Brenot, R., 2011, 10 Gbit/s modulation of
reflective SOA without any electronic processing, In
PROCEEDING’11, OFC 2011, paper OThT2.
Schmidt, B. J. C., Lowery, A. J., Armstrong, J., 2008,
Experimental demonstrations of electronic dispersion
compensation for long-haul transmission using direct-
detection optical OFDM, Journal of Lightwave
Technology, 26(1), pp. 196-203.
Chow, P. S., Cioffi, J. M., Bingham, J. A. C., 1995, A
practical discrete multitone transceiver loading
algorithm for data transmission over spectrally shaped
channels, IEEE Transactions on Communications,
43(234), pp. 773-775.
0 2 4 6 8 10 12 14 16 18 20
10
12
14
16
18
20
22
24
26
28
Preamp Input Power Difference(dB)
Maximum Data Rate(Gb/s)
Local Fed - High Ch
Local Fed - Low Ch
Remote Fed - High Ch
Remote Fed - Low Ch
Remote Fed - Measured
Local Fed - Measured
RSOA input optical power : -7 dBm
OPTICS 2012 - International Conference on Optical Communication Systems
402