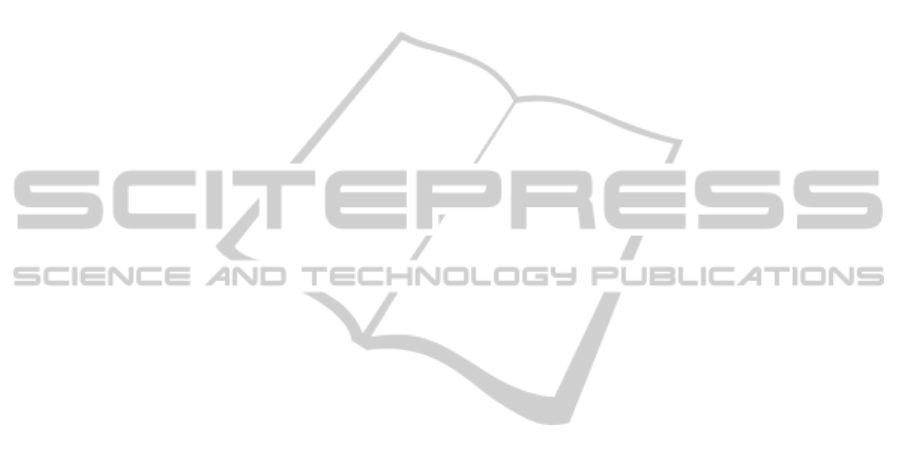
evaluating the shape of the robot and the movable
area by heights, the robot can select the traveling
direction to go through between the obstacles
keeping the safe distance from the obstacles without
unnecessary rotational motion like situation B. In
situation D shown in Figure 3(d), the height of
obstacles is higher than that of the robot arms. If the
shape of the robot at the height 1 is horizontally long
according to the baggage, the robot can go through
between the obstacles by rotational motion as well
as situation B. By contrast, in situation D that the
robot closes arms according to the size of the
baggage, the robot can select the traveling direction
to go through between the obstacles without
unnecessary rotational motion keeping the safe
distance from obstacles by evaluating the shape of
the robot by heights.
These results showed that it is possible for the
robot to select the traveling direction that makes a
short route to the goal keeping the safe distance from
obstacles by the evaluation of the shape of the robot
and the movable area by heights.
3.2 Effectiveness of Consideration of
Moving Parts
In situation E shown in Figures 4(a) and 4(c), the
robot cannot get to the goal because it is difficult to
determine the traveling direction uniquely only
using reactive motion control method in situation
that the surrounding environment of the robot is
completely symmetrical. By contrast, in situation F,
the robot changed the position of the moving part.
As shown in Figures 4(b) and 4(c), the robot can
deal with this problem by changing the relation
between the robot and the surrounding environment
depending on the position of the moving parts.
These results show that the robot becomes
possible to respond flexibly to various situations by
the proposed robot model because the choices of
method for motion control to change the relation
between the robot and the environment increase.
4 CONCLUSIONS
In this paper, toward the realization of motion
control for autonomous mobile robots that can be
flexible in various situations, the robot model
considering moving parts has been proposed. In
addition to translational and rotational motion, using
this robot model, it was verified that the changes of
the moving parts can change the relation between
the robot and the environment. Furthermore, the real
time collision avoidance method based on the fuzzy
potential method considering the shape of the robot
and the movable area by heights has been proposed.
Evaluating of the robot and the movable area for
each height, if the robot is unable to move keeping a
safe distance from the obstacles, the robot
determines the suitable orientation angle considering
the minimum length from the center of the robot
model to that outer shape. The effectiveness has
been verified by numerical simulations. It has been
shown that the robot becomes possible to respond
flexibly to various situations by proposed robot
model and control method.
REFERENCES
Tiejun, Z., Dalong, T. and Mingyang, Z. (2005). The
Development of a Mobile Humanoid Robot with
Varying Joint Stiffness Waist, Proceedings of the
IEEE International Conference on Mechatronics and
Automation, Vol. 3, pp.1402-1407.
Kuindersma, S., Hannigan, E., Ruiken, D. and Grupen, R.
(1999). MINERVA: Dexterous Mobility with the
uBot-5 Mobile Manipulator, Proceedings of the IEEE
International Conference on Robotics and Automa-
tion, Vol. 3, pp. 1999-2005.
Mehling, J. S., Strawser, P., Bridgwater, L., Verdeyen, W.
K. and Rovekamp, R. (2007). Centaur: NASA’s
Mobile Humanoid Designed for Field Work, IEEE
International Conference on Robotics and Automa-
tion, pp. 2928-2933.
Borenstein, J. and Koren, Y. (1991). The Vector Field
Histogram Fast Obstacle Avoidance for Mobile
Robots, IEEE Transactions on Robotics and Automa-
tion, Vol.7, No.3, pp.278-288.
Fox, D., Burgard, W. and Thrun, S. (1997). The Dynamic
Window Approach to Collision Avoidance, IEEE
Robotics and Automation, Vol. 4, No. 1, pp.1-23.
Minguez, J. and Montano, L. (2004). Nearness diagram
(ND) Navigation: Collision Avoidance in Trouble-
some Scenarios, IEEE Transactions on Robotics and
Automation, Vol. 20, No. 1, pp. 45-59.
Kavraki, L. (1995). Computation of Configuration Space
Obstacles Using the Fast Fourier Transform, IEEE
Transactions on Robotics and Automation, Vol. 11,
No. 3, pp. 408-413.
Wang, Y. and Chirikjian, G. S. (2000). A New Potential
Field Method for Robot Path Planning, Proceedings
IEEE International Conference on Robotics and Auto-
mation, San Francisco, CA, pp. 977-982.
Suzuki, T. and Takahashi, M. (2011). Translational and
Rotational Motion Control Considering Width for
Autonomous Mobile Robots Using Fuzzy Interence,
Numerical Analysis – Theory and Application, InTech
Book, ISBN 978-953-307-389-7.
Tsuzaki, R. and Yoshida, K. (2003). Motion Control
Based on Fuzzy Potential Method for
AutonomousMobile Robot with Omnidirectional
Vision. Journal of the Robotics Society of Japan,
Vol.21, No.6, pp.656-662.
ICINCO 2012 - 9th International Conference on Informatics in Control, Automation and Robotics
454