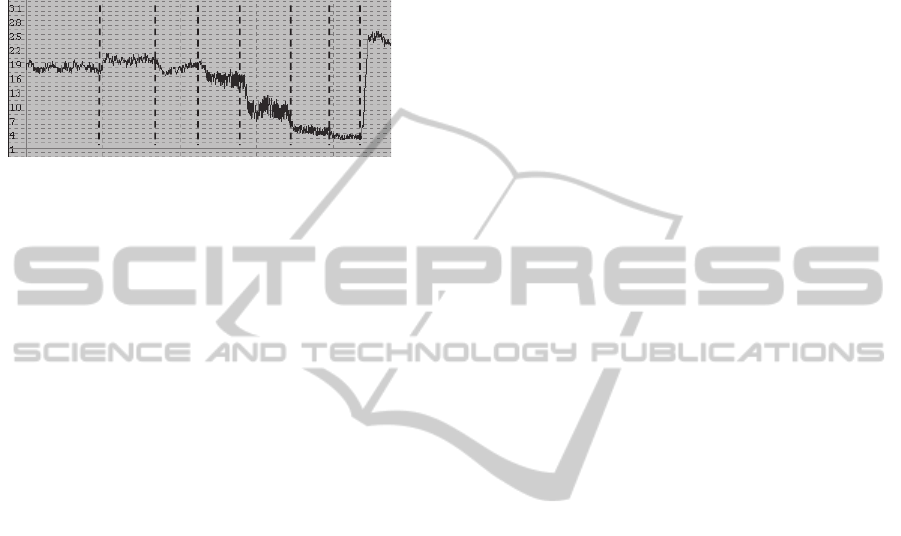
used LDF diagnostic technique and calibrated
cargoes which were put on a tip of the probe. An
example of continuous recording of the blood
microcirculation index I
m
in the finger pulp skin at
different probe pressure levels is presented in figure
9.
a b c d e f g
Figure 9: Registered blood flow at different pressure: (a)
without pressure, (b) 0.6 G/mm
2
; (c) 1.2 G/mm
2
; (d) 2.3
G/mm
2
; (e) 8.6 G/mm
2
; (f) 10.2 G/mm
2
; (g) 14.9 G/mm
2
.
The diagram shows that the pressure of around
10 G/mm
2
almost completely blocks the blood flow
in the finger skin. The more pressure is increased the
more different fluctuations in I
m
arise within the
pressure interval 2-10 G/mm
2
. In a similar way the
pressure impacts both on TRO and LFS data,
because under pressure the blood is squeezed out of
the microvasculature, and light absorption by blood
is decreased in the DV. The changes in absorption
determine a maximum of registered I
m
in the b
interval of the pressure. Thus, the interaction of a
surveyed biological object and an optical fiber probe
can lead to dramatic instrumental errors without
appropriate pressure control. More than 50% of the
total error according to our data can be accounted by
the interactive component of the error.
4 CONCLUSIONS
In the present study we have investigated some main
sources of errors and uncertainties in diagnostic data
caused by using an optical multi-fibers probe in
NMS. With the use of well-known statistical
methodology of multiple-repeated measurements
both on lifeless imitational tissue-like phantoms and
on real clinical patients (volunteers) the dispersion
and errors up to a level of +/-36,3% of the average
registered quantities were discovered in NMS. It is
shown that the interactive component of errors
caused by interaction of the optical fiber probe and
surveyed object gives the main contribution to the
total uncertainty of the measurement results.
The direct comparison of the diagnostic data
when using different probes with different distances
between fibers in the cord shows that for each DV
the constructive parameters of each optical fiber
probe should be standardised to have comparable
results. Also, a professional qualification of doctors
as well as a precisely formulated diagnostic route of
the inspection of patients are important components
of actions to reduce errors in NMS.
REFERENCES
Jenny, G., Biallas, M., Trajkovich, I., Fauchere, J-C.,
Bucher, H., Wolf, M., 2011. Reproducibility of
cerebral tissue oxygen saturation measurements by
near-infrared spectroscopy in newborn infants. J.
Biomed. Opt. 16. 097004.
Kutai-Asis, H., Kanter, I., Barbiro-Michaely E.,
Mayevsky, A., 2008. Classification of tissue
pathological state using optical multiparametric
monitoring approach. Proc. SPIE. 7280. 72801K.
Lim, L., Nichols, B., Rajaram, N., Tunnell, J., 2011. Probe
pressure effect on human skin diffuse reflectance and
fluorescence spectroscopy measurements. J. Biomed.
Opt. 16. 011012.
Nishidate, I., Maeda T., 2007. Visualizing depth and
thickness of a local blood region in skin tissue using
diffuse reflectance images. J. of Biomed. Opt., 12(5),
054006.
Pochivalik, M., Pichler, G., Zotter, H., Tax, N., Muller,
W., Urlesberger, B., 2011. Regional tissue oxygen
saturation: comparability and reproducibility of
different devices. J. of Biomed. Opt., 16, 057004.
Reif, A., Amorosino, M., Calabro, K., A’Amar, O., Singh,
S., Bigio, I., 2008. Analysis of changes in reflectance
measurements on biological tissues subjected to
different probe pressures. J. Biomed. Opt. 13(1).
010502.
Rogatkin, D., Prisnyakova, O., Moiseeva, L., 1998.
Analysis of the accuracy of clinical laser fluorescence
diagnosis. Measurement Techn. 41(7), 670-674.
Rogatkin, D., Lapaeva, L., 2003. Prospects for
development of noninvasive spectrophotometric
medical diagnosis. Biomed. Engin. 37(4). 217-222.
Rogatkin, D., Lapaeva, L., Petritskaya, E., Sidorov, V.,
2009. Multifunctional laser noninvasive spectroscopic
system for medical diagnostics and metrological
provisions for that. Proc. SPIE. 7368. 73681Y.
Rogatkin, D., Dunaev, A., Lapaeva, L., 2010.
Metrological support of methods and devices for non-
invasive medical spectrophotometry. Biomed.
Engineering. 44(2). 66-70.
Tchernyi, V., Rogatkin, D., Gorenkov, R. Karpov, V.,
Shumskiy, V., 2006. Complex noninvasive
spectrophotometry in examination of patients with
vibration disease. Proc. SPIE. 6078. 60782.
BIODEVICES2013-InternationalConferenceonBiomedicalElectronicsandDevices
238