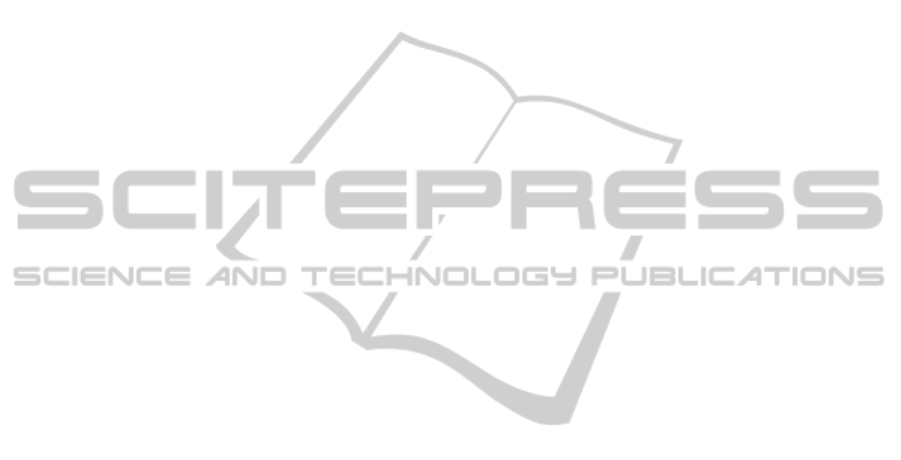
From the three subjects who experienced thermal
stimuli in NP group, only one presents complete
injury. For the P group, two subjects are AIS B and
five are AIS A.
This finding can be justified through theories that
explain why subjects with complete SCI have some
sensibility, characterizing the discomplete injury.
Dimitrijevic (1988) and Sherwood, Dimitrijevic and
Mckay (1992) found motor remnants in complete
SCIS due to a neural control. Thus, discomplete
injury is an incomplete injury that fits the AIS
criteria for grade A. Moreover, some subjects with
complete SCI can present some semblance of
sensibility, which can be evoked below the level of
injury due to incomplete injuries in the
spinothalamic tract. These subjects have subclinical
functions of ascending and descending tracts
(Finnerup et al., 2004).
5 CONCLUSIONS
Due to feedback control, the custom built portable
device provides easy temperature control with
resolution of 1
o
C. The device is simple to build and
can stabilize its temperature in about 5s for a 5
o
C
temperature change, therefore representing a simple
alternative for quick and practical assessment. It can
provide quantified information about sensory
performance of subjects, and the results obtained
from clinical trials are in accordance to previous
works, thus demonstrating the device feasibility for
thermal sensitivity assessment. Spinal cord injured
subjects that refer neuropathic pain are more
sensitive to thermal stimuli than patients do not
present neuropathic pain.
ACKNOWLEDGEMENTS
We thank the support by grants from São Paulo
Research Foundation (FAPESP) and National
Council for Scientific and Technological
Development (CNPq).
REFERENCES
Bonica, J. J., 1991. Introduction: semantic, epidemiologic,
and educational issues. In: Casey, K. L. Pain and
Central Nervous System Disease: the Central Pain
Syndromes. Raven Pr. New York: Raven Pr, pp 13–
29.
Callister Jr., W. D., 2001. Fundamentals of Material
Science and Engineering. 5
th
ed. New York: John
Wiley & Sons, Inc.
Catafau, S., Bosque, Q., 2003. Mecanismos
fisiopatológicos da dor neuropática, 1
st
ed. Madrid:
Medica Panamericana.
Dahlberg, A., Alaranta, H., Sintonen, H., 2005. Health-
related quality of life in persons with traumatic spinal
cord lesion in Helsinki. Journal of Rehabilitation
Medicine, 37, pp. 312–316.
Dimitrijevic, M. R., 1988. Residual motor functions in
spinal cord injury. Advances in Neurology, 47, pp.
138–155.
Eng, J. J., Miller, W. C., 2006. Rehabilitation: from
bedside to community following spinal cord injury
(SCI). In: Eng, J. J., Teasell, R. W., Miller, W. C.,
Wolfe, D. L., Townson, A. F., Aubut, J., Abramson,
C., Hsieh, J. T. C., Connolly, S. Spinal Cord Injury
Rehabilitation Evidence. Vancouver, pp. 16–29.
Finnerup, N. B., Johannesen, I. L., Fuglsang-Frederiksen,
A., Bach, F. W., Jensen, T. S., 2003. Sensory function
in spinal cord injury patients with and without central
pain. Brain, 126, pp. 57–70.
Finnerup, N. B., Gyldensted, C., Fuglsang-Frederiksen,
A., Bach, F. W., Jensen, T. S., 2004. Sensory
perception in complete spinal cord injury. Acta
Neurologica Scandinavica, 109, pp. 194–199.
Kaplan, S. A., Chancellor, M. B., Blaivas, J. G., 1991.
Bladder and sphincter behavior in patients with spinal
cord lesions. Journal of Urology, 146, pp. 113–117.
Kenshalo, D. R., Bergen, D. C., 1975. A device to
measure cutaneous temperature sensitivity in humans
and subhuman species. Journal of Applied Physiology,
39, pp. 1038–1040.
Kirillova, I., Rausch, V. H., Baron, R., Jänig, W., 2011.
Mechano- and thermosensitivity of injured muscle
afferents. Journal of Neurophysiology, 105, pp. 2058–
2073.
Kirshblum, S. C., Burns, S. P., Biering-Sorensen, F.,
Donovan, W., Graves, D. E., Jha, A., Johansen, M.,
Jones, L., Krassioukov, A., Mulcahey, M. J., Schmidt-
Read, M., Waring, W. 2011. International standards
for neurological classification of spinal cord injury
(Revised 2011). The Journal of Spinal Cord Medicine,
34, pp. 535–546.
Maynard Jr., F. M., Bracken, M. B., Creasey, G., Ditunno
Jr, J. F., Donovan, W. H., Ducker, T. B., Garber, S. L.,
Marino, R. J., Stover, S. L., Tator, C. H., Waters, R.
L., Wilberger, J. E., Young, W., 1997. International
standards for neurological and functional classification
of spinal cord injury. Spinal Cord, 35, pp. 266–274.
Melzack, R., 1975. The McGill Pain Questionnaire: major
properties and scoring methods. Pain, 1, pp. 277–299.
Melzack, R., Torgerson W. S., 1971. On the language of
pain. Anesthesiology, 422, pp. 50–59.
Nomoto, S., Shibata, M., Iriki, M., Riedel, W., 2004. Role
of afferent pathways of heat and cold in body
temperature regulation. International Journal of
Biometeorology, 49, pp. 67–85.
Pimenta, C. A. de M., Teixeira, M. J., 1996. Questionário
PortableCustomBuiltDeviceforThermalSensitivityAssessment-AnAuxiliaryTooltoCharacterizetheNeuropathic
PainfollowingSpinalCordInjury
33