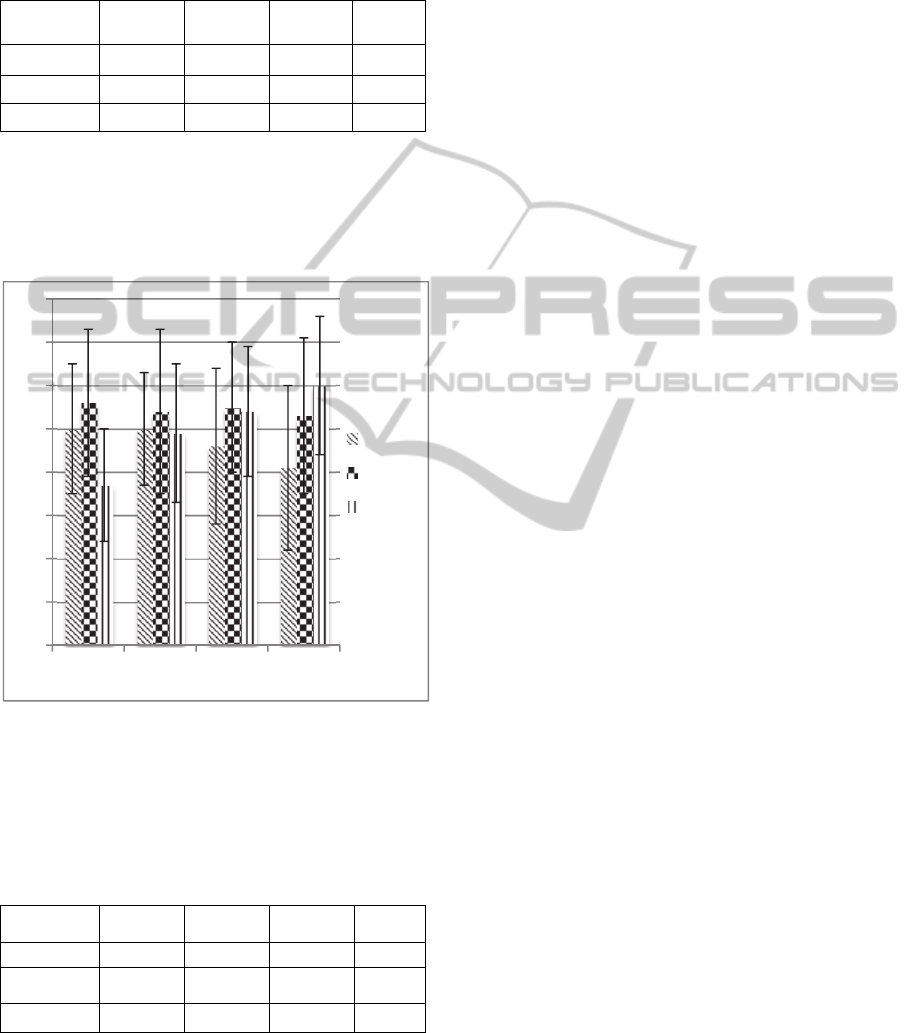
autonomic neuropathy in both supine and tilt-up
positions were significantly larger than that of
diabetics with autonomic neuropathy.
Table 4: Max CCF values at LF in each group.
LF
Max CCF
NL DM DAN1 DAN2
Supine 0.52±0.09 0.48±0.08 0.42±0.09 0.45±0.1
Tilt-up 0.6±0.13 0.59±0.13 0.46±0.08 0.48±0.16
HV 0.45±0.08 0.43±0.1 0.4±0.09 0.41±0.11
The results were especially significant in tilt-up
position. It indicates that the cerebral autoregulation
(CA) of normal subjects and diabetics without
autonomic neuropathy is superior to that of diabetics
with autonomic neuropathy.
Figure 1: Comparison of Max CCF values at VLF in each
group.
Max CCF values at LF of normal subjects and
patients with different autonomic neuropathy are
shown in Table 5.
Table 5: Max CCF values at VLF in each group.
VLF MAX
CCF
NL DM DAN1 DAN2
Supine 0.5±0.15 0.5±0.13 0.46±0.18 0.41±0.19
Tilt-up 0.56±0.17 0.54±0.19 0.55±0.15 0.53±0.18
HV 0.56±0.13 0.49±0.16 0.54±0.15 0.6±0.16
Max CCF values gradually increased in
hyperventilation at VLF from normal subjects to
diabetics without autonomic neuropathy, diabetics
with mild autonomic neuropathy, and diabetics with
severe autonomic neuropathy shown in Figure 1. It
reveals that the diabetes have worse vascular
compliance in comparison with the normal subjects.
4 CONCLUSIONS
In this study, the power spectral density (PSD)
analysis and cross-correlation function (CCF)
analyses were applied to demonstrate
hyperventilation can help us to assess the normal
subjects and diabetics with various degrees
autonomic neuropathy. On the contrary, the opposite
results could be obtained in tilt-up position.
ACKNOWLEDGEMENTS
The authors would like to thank the National
Science Council, Taiwan, R.O.C., for supporting this
research under Contract No. NSC-99-2221-E-035-
054-MY3.
REFERENCES
Lassen, N. A., 1959, Cerebral Blood Flow and Oxygen
Consumption in Man, Physiological Reviews, Vol. 39,
pp. 183-238.
Mitsis, G. D, Zhang, R., Levine, B. D., Tzanalaridou, E.,
Katritsis, D. G., and Marmarelis, V. Z., 2009.
Autonomic Neural Control of Cerebral
Hemodynamics, IEEE Engineering in Medicine and
Biology Magazine, Vol. 28, pp. 54-62.
Panerai, R. B., Simpson, D. M., Deverson, S. T., Mathony,
P., Hayes, P., and Evans, D. H., 2000. Multivariate
Dynamic Analysis of Cerebral Blood Flow Regulation
in Humans, IEEE Transactions on Biomedical
Engineering, Vol. 47, pp. 419-423.
Blaber, A. P., Bondar, R. L., Stein, F., Dunphy, P. T.,
Moradshahi, P., Kassam, M. S., Freeman, R., 1997,
Transfer function analysis of cerebral autoregulation
dynamics in autonomic failure patients, Stroke, Vol.
28, pp. 1686-1692.
Chiu, C. C. and Yeh, S. J., 2001. Assessment of cerebral
autoregulation using time-domain cross-correlation
analysis, Computers in Biology and Medicine, Vol. 31,
No. 6, pp. 471-480.
Chiu, C. C., Yeh, S. J., and Liau, B. Y., 2005, Assessment
of Cerebral Autoregulation Dynamics in Diabetics
Using Time-domain Cross-correlation Analysis,
Journal of Medical and Biological Engineering, Vol.
25, pp. 53-59.
0
0,1
0,2
0,3
0,4
0,5
0,6
0,7
0,8
NL DM DAN1 DAN2
Supine
Tilt‐up
HV
QuantitativeAssessmentofDiabeticswithVariousDegreesofAutonomicNeuropathy
305