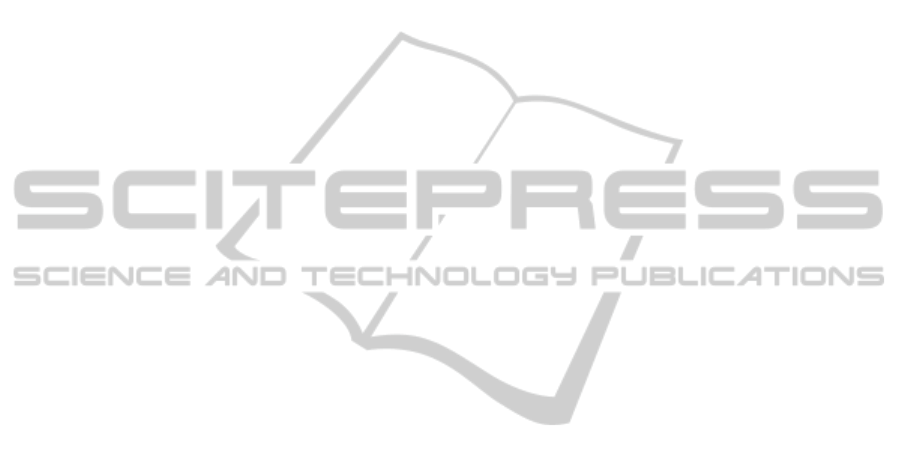
materials. There are a number of candidates for
biocompatible and biopassive surfaces. For example,
organosilicone coatings are electrically insulating
and biopassive.
Poly(trivinyltrimethylcyclotrisiloxane) (PV
3
D
3
)
films have been deposited by using iCVD
(O’Shaughnessy et al., 2006); (Murthy et al., 2002).
PV
3
D
3
has a highly crosslinked structure that makes
it insoluble in both polar and non-polar solvents.
This makes the structure also very stable. Another
possible monomer is Poly (tetrafluoroethylene)
(PTFE). It has been found that the rate of deposition
of PTFE films is dramatically increased with the
addition of perfluorooctane (PFOS) in the gaseous
mixture (Baxamusa et al., 2009). PTFE films can be
conformally coated. They are also hydrophobic and
biocompatible and are used in the medical device
industry and hence are expected to be a good
candidate material as a nanobattery packaging
system.
The iCVD films can be deposited rapidly and the
technology is scalable. Hence, iCVD is a very
attractive technique for packaging of nanobatteries
and any other nanoscale medical devices.
3 CONCLUDING REMARKS
In this work we report the fabrication of micro- and
nano-batteries using femtosecond laser machining.
Though the first batteries are array of n x n micro-
cells, laser machining is clearly a technology that
would allow the fabrication of a single nanobattery.
Though the field of nanobatteries is still in its
infancy, we have outlined methods that should lead
to the fabrication of an array of nanobatteries or
even a single nanobattery appropriately packaged in
biopassive and biocompatible coatings. This, then,
could be the first step to the development of power
sources for future nanodevices that can be directly
implanted in biological systems.
ACKNOWLEDGEMENTS
The authors would like to thank the National
Science Foundation and Missouri State University
for financial support.
REFERENCES
Baxamusa, S. H.; Im, S. G.; and Gleason, K., 2009.
“Initiated and oxidative chemical vapor deposition a
scalable method for conformal and functional polymer
films on real substrates”, Phys. Chem. Chem. Phys. 11,
5227 – 5240.
Brazier, A.; Dupont L.; Dantras-Laffont L.; Kuwata, N.;
Kawamura, J.; Tarascon, J.-M., 2008. “First cross-
section observation of an all solid-state lithium-ion
“nanobattery” by transmission electron microscopy”,
Chem. Mater. 20, 2352-2359.
Dewan, C. and Teeters, D., 2003. “Vanadia xerogel
nanocathodes used in lithium microbatteries”, J.
Power Sources 119-121, 310-315.
Dudney, N. J., 2000. “Addition of thin-film organic solid
electrolytes (Lipon) as protective film in lithium
batteries with liquid electrolyte”,“J. Power Sources
89, 176.
Humble, P. H. and Harb, J. N., 2003. “Optimization of
Nickel-Zinc microbatteries for hybrid powered
microsensor systems”, J. Electrochem. Soc. 150, 1182.
Humble, P. H.; Harb, J. N.; Lafolette, R., 2001.
“Microscopic nickel-zinc batteries for use in
autonomous microsystems”, J. Electrochem. Soc. 148,
1357.
Jogelkar, A. P.; Liu, H.-H.; Meyhofer, E.; Mourou, G.;
and Hunt, A. J., 2004 “Optics at Critical Intensity:
Applications to Nanomorphing”, PNAS, 101, 5856-
5861.
Jogelkar, A. P.; Liu, H.-H.; Spooner, G.; Meyhofer, E.;
Mourou, G.; and Hunt, A., 2003. J. Appl. Phys. B. 77,
23-30.
Layson, A. L.; Gadad, S.; and Teeters, D., 2003.
Electrochim. Acta 48, 2207.
Murthy, S. K.; Olsen, B. D. and Gleason, K. K., 2002.
“Initiation of cyclic vinylmethylsiloxane
polymerization in a hot-filament chemical vapor
deposition process”, Langmuir 18, 6424–6428.
O’Shaughnessy, W. S.; Gao, M. L. and Gleason, K. K.,
2006. “Ínitiated chemical vapor deposition of
trivilylmethylcyclotrisilozane for biomaterial
coatings”, Langmuir, 22, 7021–7026.
Park ,Y. J.; Kim, J. G.; Kim, M. K.; Kim, H. G.; Chung,
H. T.; Park, Y., 2000. “ Electrochemical properties of
LiMnO thin films: 24 suggestion of factors for
excellent rechargeability”, J. Power Sources 87, 69.
Park ,Y. J.; Park, K. S.; Kim, J. G.; Kim, M. K.; Kim, H.
G.; Chung, H. T., 2000. “Characterization of tin
oxide/LiMn
2
O
4
thin-film cell”, J. Power Sources 88,
250.
Squier, J.; Salin, F.; Mourou, G.; and Her, H. H., 1991.
“100-fs Pulse Generation and amplification in
Ti:Al
2
O
3
”, Opt. Lett. 16, 324-327.
Stephan, A. M. and Teeters, D., 2003. “Characterization of
PVdF-HFP polymer electrolytes confined in micor and
nanopores”, Electrochim. Acta 48, 2143.
Stuart, B. C.; Feit, M. D.; Herman, S., 1996. “Effect of
polarization on ultrashort pulsed laser ablation of thin
metal films”, J. Opt. Soc. Am, B 13, 459..
Venkatakrishnan, K.; Tan, B.; Stanley, P.; and Sivakumar,
N. R., 2002. “The effect of polarization on ultrashort
pulsed laser ablation of thin metal films”, J. Appl.
BIODEVICES2013-InternationalConferenceonBiomedicalElectronicsandDevices
130