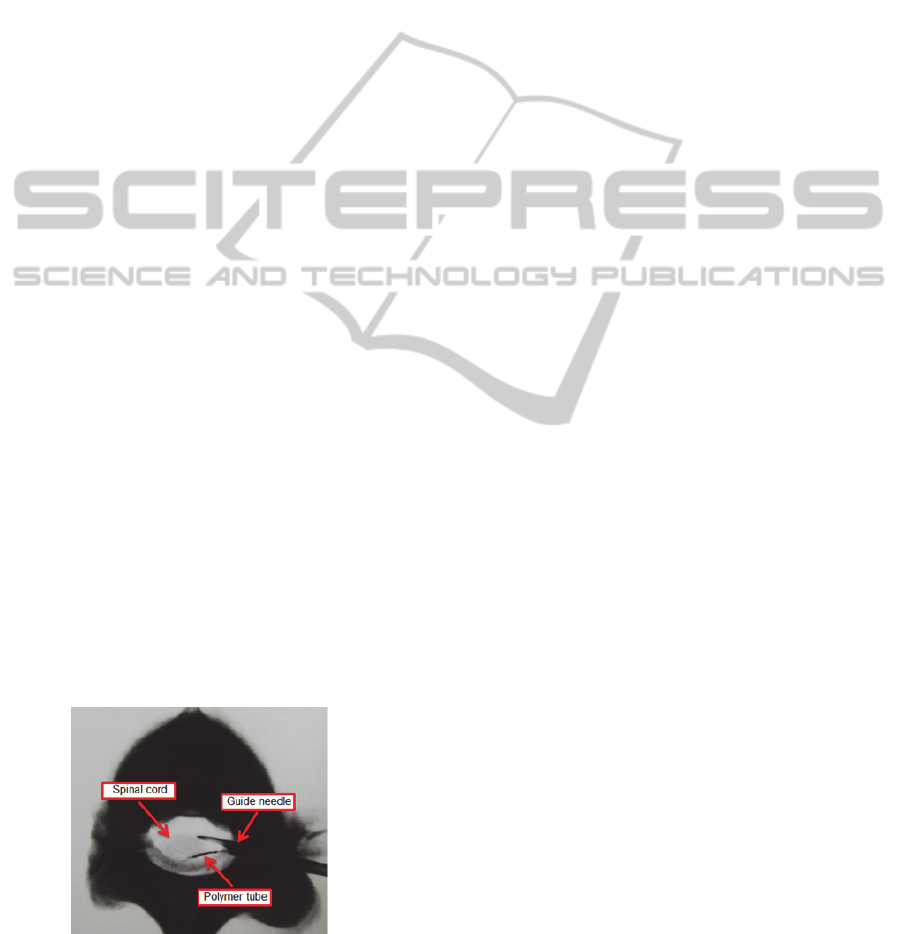
present intradiscal microprobe is thought to be high
enough to provoke pains because the method
directly irritates the nerve ending, not the bone.
In addition, the preliminary experiment to
evaluate the ex vivo steering performance of the
intradiscal microprobe was implemented using C-
arm x-ray imaging. The experiment was carried out
in a pig spinal cord, instead of the nucleus pulposus,
because an x-ray image of the polymer tube would
not be visible due to the opaque vertebrae located at
top and bottom sides of the nucleus pulposus.
Considering that the mechanical properties of the
jelly-like spinal cord are similar to those of the
nucleus pulposus, this preliminary experiment is
thought to be able to provide meaningful data for
subsequent ex vivo experiment.
Prior to the ex vivo experiment, we conducted a
steering test of the polymer tube in air to compare
with the steering performance in the ex vivo spinal
cord. The protruding length of the polymer tube,
from the guide needle, was decreased to 13 mm,
because the diameter of the pig spinal cord prepared
for the experiment was only 15 mm. The maximum
steering angle of the 13 mm length of the protruding
tube in air was measured to be 42°, which is about
half that of the 30 mm protruding tube. The
reduction in the steering angle is attributed to the
shortening of the protruding tube length, which is
easily predictable.
Fig. 6 is an ex vivo x-ray image (C-arm) which
shows that the polymer tube was successfully guided
to the outer rim of the pig spinal cord. The
maximum steering angle in the spinal cord was
measured as 40°, which is in good agreement to that
in air. This indicates that there is a negligible
difference in the steering angle in air and that in the
spinal cord. Even in a case of the tube protruding 30
mm, we can infer that the steering angle and
amplitude in clinical trials will be almost the same as
the results obtained from experiments in air.
Figure 6: The C-arm picture of the polymer tube steered
towards the outer rim of the pig spinal cord.
In a further study, we will carry out an in vitro
vibration experiment using a model that has
mechanical properties similar to those of the nucleus
pulposus before clinical trials. Also, a high-
performance linear motor will be used to achieve a
wider range of vibration frequency and amplitude.
ACKNOWLEDGEMENTS
This research was supported by a grant from the
Institute of Medical System Engineering (iMSE) of
the Gwangju Institute of Science and Technology
(GIST), Republic of Korea.
REFERENCES
Yrjama, M. and Vanharanta, H., 1994, Bony Vibration
Stimulation: a New, Non-Invasive Method for
Examining Intradiscal Pain. European Spin Journal,
3:233-235.
Burke, J. G., Watson, R. W. G., McCormack, D., Dowling,
F. E., Walsh, M. G. and Fitzpatrick, J. M., 2002.
Intervertebral Discs Which Cause Low Back Pain
Secrete High Levels of Proinflammatory Mediators.
Journal of Bone and Join Surgery, Vol.84-B:196-201.
Schwarzer, A. C., Aprill, C. N., Derby, R., Fortin, J., Kine,
G. and Bogduk, N., 1995. The Prevalence and Clinical
Features of Internal Disc Disruption in Patients With
Chronic Low Back Pain. Spine, Vol.20:1878-1883.
Shin, D., Kim, H., Jung, J., Shin, D. and Lee, J., 2006,
Diagnostic Relevance of Pressure-Controlled
Discography, Journal of Korean Neurosurgical
Society, 21(5):911-916.
Kim, H., Shin, D., Kim, H., Yoo, E., Shin, D. and Lee, J.,
2009, Clinical and Radiological Findings of
Discogenic Low Back Pain Confirmed by Automated
Pressure-Controlled Discography. Journal of Korean
Neurosurgical Society, 46(4):333-339.
Adams, M. A., Dolan, P. and Hutton, W. C., 1986, The
Stages of Disc Degeneration as Revealed by
Discograms. Journal of Bone and Joint Surgery,
Vol.68-B:36-41.
BIODEVICES2013-InternationalConferenceonBiomedicalElectronicsandDevices
162