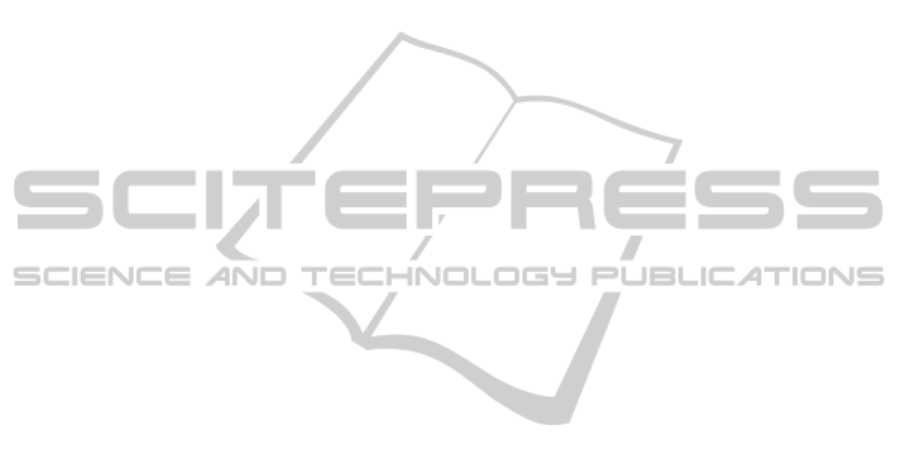
head was 2 m. The head was mounted on a pan-tilt
unit. The sampling rate was 44.1 kHz and the sounds
had different durations. Simple omnidirectional web-
cam microphones were mounted in the head at the ear
positions (without pinnae). All experiments were re-
peated 7 times at 180 distinct positions in front of the
head (-90
◦
to +90
◦
in 1
◦
steps).
Figure 4 shows the results obtained with the
dummy head. Between -30
◦
and +30
◦
, the average
error is less than 1
◦
for all sounds. Beyond ±30
◦
the
error is bigger, on average 2.73
◦
with a maximum er-
ror of about 15
◦
. Interestingly, the results of the high-
frequency whistle sound (5-7 kHz) are comparable to
those of the low-frequency sounds. At high frequen-
cies, auditory nerves do not phase-lock and angle es-
timation is based on projections of ITD-env sensitive
cells in the IC. With the dummy head the model could
differentiate ILDs from -60
◦
to +60
◦
. ILD-only re-
sults were reliable with an accuracy of 15
◦
.
The KEMAR HRTF database is available with a
resolution of 5
◦
. Therefore the peaks of the ITD func-
tions of V-type neurons were re-trained using these
angles. Since the resolution is less, and the HRTFs
were measured in an anechoic chamber, we expected
a better accuracy. Indeed, the results obtained are very
accurate between ±40
◦
. Beyond these angles, accu-
racy decreases: the mean error was 2.1
◦
and the maxi-
mum error was only 5
◦
. ILD-only resolution was also
15
◦
from -60
◦
to +60
◦
.
5 CONCLUSIONS
In this paper we described a computational model
based on the mammalian brain. It employs ITD, ILD
and ITD-env detection pathways from the cochlear
nuclei to the inferior colliculus. V-type neurons in
the IC with peak-type ITD functions are used. Their
distribution in the IC and their input projections from
MSO and DNLL are modeled. I-type neurons are
simulated to determine ILD and ITD-env. All inter-
aural cues are combined in the IC to yield broad-
band sound source localization. The merging of the
cues yields a reliable and accurate system that works
in the human frequency range. Experimental results
were very good. In the future, azimuthal localization
will be complemented by the elevation angle, using
ear-like pinnae and type-O neurons sensitive to spec-
tral notches. The connection of the IC to the motor-
sensory pathways and hippocampus will be investi-
gated to move a robot toward sounds. Audio-visual
object localization and identification is a particular
field of interest.
ACKNOWLEDGEMENTS
Pluri-annual funding of ISR/LARSyS by the Por-
tuguese Foundation for Science and Technology
(FCT), and EU project NeuralDynamics, FP7-ICT-
2009-6, PN: 270247.
REFERENCES
Blauert, J. (2001). Spatial Hearing. MIT Press.
Fitzpatrick, D. C., Batra, R., Stanford, T. R., and Kuwada,
S. (1997). A neuronal population code for sound lo-
calization. Nature, 388(6645):871–874.
Gardner, B. and Martin, K. (2000). HRTF Measurements
of a KEMAR Dummy-Head Microphone. MIT Media
Lab.
Jeffress, L. A. (1948). A place theory of sound localization.
J Comp Physiol Psychol, 41(1):35–9.
Liu, J., Perez-Gonzalez, D., Rees, A., Erwin, H., and
Wermter, S. (2010). A biologically inspired spik-
ing neural network model of the auditory midbrain
for sound source localisation. Neurocomputing,
74(13):129–139.
Palmer, A. and Kuwada, S. (2005). Binaural and spa-
tial coding in the inferior colliculus. In : Winer, J.
and Schreiner, C. (eds), The Inferior Colliculus, pages
377–410. Springer New York.
Ramachandran, R. and May, B. J. (2002). Functional seg-
regation of itd sensitivity in the inferior colliculus of
decerebrate cats. J Neurophysiol, 88(5):2251–61.
Raspaud, M., Viste, H., and Evangelista, G. (2010). Bin-
aural source localization by joint estimation of ild and
itd. Trans. Audio, Speech and Lang. Proc., 18(1):68–
77.
Rose, J. E., Gross, N. B., Geisler, C. D., and Hind, J. E.
(1966). Some neural mechanisms in the inferior col-
liculus of the cat which may be relevant to localization
of a sound source. J Neurophysiol, 29(2):288–314.
Slaney (1993). An efficient implementation of the
Patterson-Holdsworth auditory filter bank. Apple
Computer Technical Report, 35.
Willert, V., Eggert, J., Adamy, J., Stahl, R., and Kaerner,
E. (2006). A probabilistic model for binaural sound
localization. IEEE Trans Syst Man Cybern B,
36(5):982–94.
Winer, J. and Schreiner, C. (2005). The central auditory
system: A functional analysis. In : Winer, J. and
Schreiner, C. (eds), The Inferior Colliculus, pages 1–
68. Springer New York.
Yin, T. C. T. (2002). Neural mechanisms of encoding bin-
aural localization cues in the auditory brainstem. In
: Fay, R.R., Popper, A.N. (eds), Integrative Functions
in the Mammalian Auditory Pathway, pages 99–159.
SpringerVerlag.
ABiologicalSoundSourceLocalizationModel
337