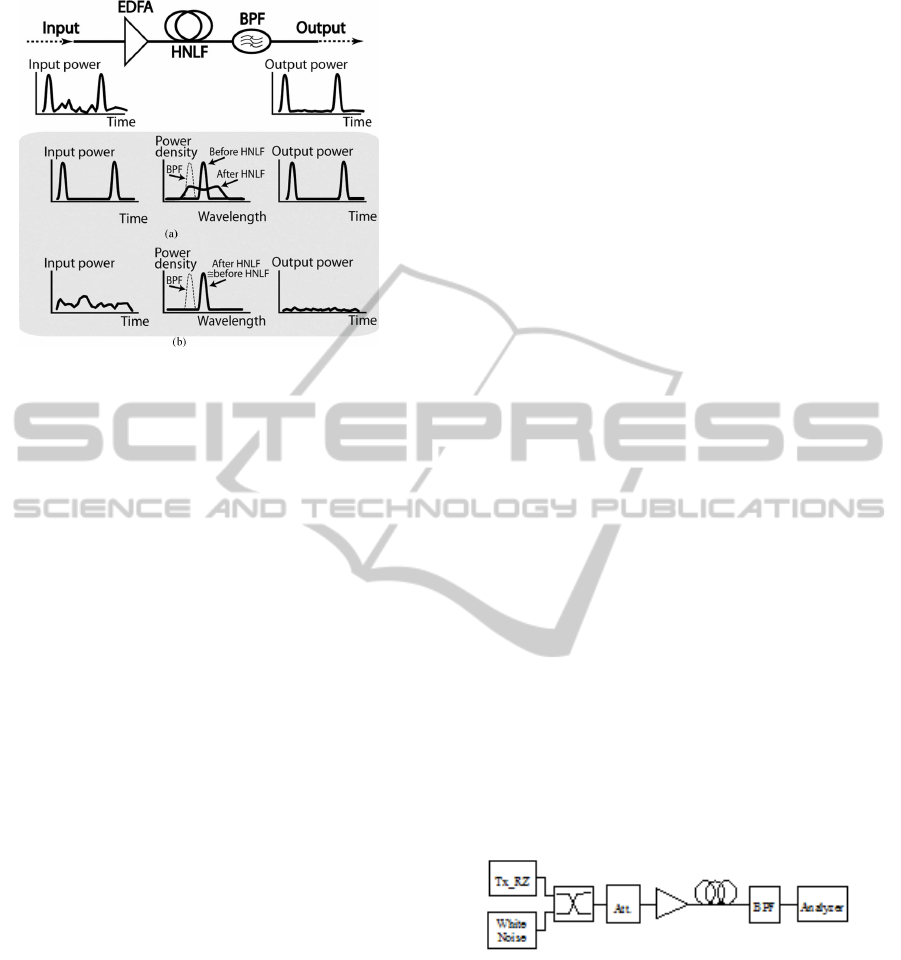
Figure 2: Block diagram of the simulated SPM
regenerator. (a) Signal and (b) noise are in the time and
frequency domain. (Rochette, Fu, Ta’eed, Moss and
Eggleton, 2006).
SPM does not change the pulse shape in the time
domain. It just causes spectrum broadening in the
frequency domain. Higher intensity causes bigger
spectral broadening as reported in Figure 1. It was
simulated with the VPI and the SPM effect was
produced by the regenerator’s nonlinear fibre
section. The blue dash curve shows the spectrum of
a pulse with 2mW power after the fibre. In this case
the fibre’s nonlinearity was turned off. Then it was
turned on, but the effect of SPM was negligible, so it
is not observable. The green curves show how the
signal’s spectrum broadening at higher pulse power
levels.
3 WORKING PRINCIPLE
SPM regenerators consist of three elements: an
amplifier, a fibre and an optical band pass filter
(Figure 2). Use of a highly nonlinear fibre is
recommended, because of the higher nonlinear effect
and it needs less power to reach its nonlinear
behaviour. The optical amplifier can be a commonly
used EDFA (Erbium Doped Fibre Amplifier) or a
SOA. It has to amplify the signal at least to a
minimum power level, which needs for the effect of
SPM to start taking place. If we reach or exceed that
power, the signal’s spectrum will be broadening by
the SPM. The regenerator’s principle of operation
(Mamyshev
, 1998) can be seen on Figure 2. The
pulse’s intensities are much higher than the noise
level and the pulses have coherent phase, while the
noise does not. Their spectrums’ shapes are very
similar, but completely different in the time domain.
The nonlinearity of the fibre will broaden the
signal’s spectrum, but the noise spectrum will not
broaden, because its power is too low for any
noticeable broadening, as we saw in Figure 1. One
piece of the broadened spectrum has to fall into the
optical band pass filter’s bandwidth, otherwise only
noise is at the output of the regenerator. The
regenerator works properly, if the whole noise’s
spectrum is filtered out by the filter. Its centre
frequency is shifted away a little bit due to the noise.
This can be critical in the DWDM (Dense
Wavelength Division Multiplexing) systems, where
the wavelength accuracy is important.
4 SIMULATIONS
The transmitter produces 2mW high RZ (return to
zero) pulses at 193.1THz with a bit rate of 0.5Gbps.
Its bit sequence was set to an alternating stream. The
signal degradation was simulated with a white noise
block and an attenuator. The white noise was added
to the signal with a coupler which introduced an
additional attenuation of 3dB. The noise was
considered Gaussian-distributed and its spectral
power density was 10
-16
W/Hz. The attenuator was
placed after the coupler. It created an overall loss of
10dB. In the regenerator a black box amplifier was
used, because only the amplifier’s gain was
important in our investigation, not the amplifying
technique. Its gain was 30dB and it also added noise
to the signal. A standard single mode fibre was used
instead of a highly nonlinear fibre, because its price
is much lower, but we had to use higher intensity for
the nonlinear effect to appear and a longer fibre
span.
Figure 3: Block scheme of the simulated regenerator.
These two disadvantages can easily eliminate the
price benefit of the standard fibre. In the simulation
the fibre’s length was 80km, which is four times
longer, than an average dispersion compensating
fibre’s length which is used in networks. An optical
filter was placed at the output of the regenerator and
an analyzer connected to its output to monitor the
signal (Figure 3). Three filter types were simulated,
which had different kind of transfer function. The
1GHz wide spectrum is broadened to 5.5GHz using
SuitableOpticalFilterforSelf-phaseModulationRegenerators
69