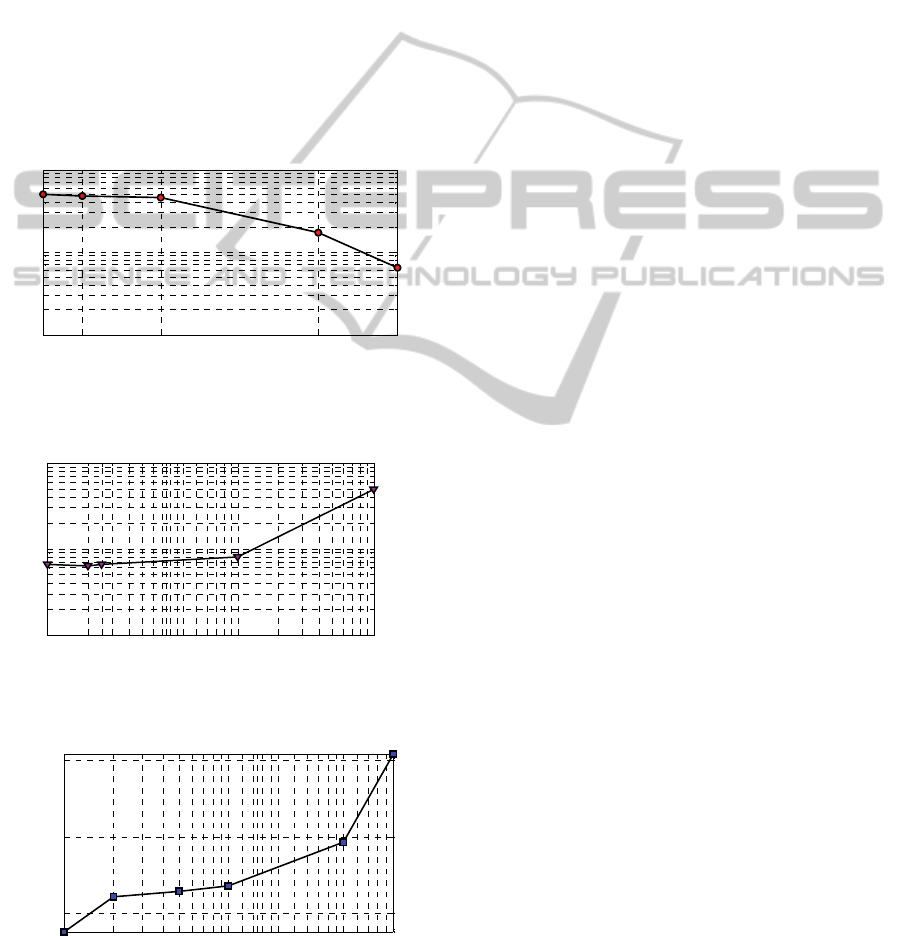
range investigated is in between 400 nm and 100
m,
which covers a wide range of TN-RN distances for
water medium as reported in (Mahfuz et al., 2010b).
Finally, the effects of data rate on BER are shown in
Fig. 7 such that BER increases as f increases. This is
also due to the ISI caused by the temporal spreading
of the channel when the input symbol changes at a
higher rate. When f increases the symbol duration
decreases and as a result the receiver cannot cope up
with the input signal to decode the transmitted bits
correctly, and in addition, suffers from the ISI. The
effects of ISI become more severe when f increases
further giving rise to BER of ~6% at f=0.01 bits per
second (bps) to ~7% at f=1 bps when r and N are
kept fixed at 800 nm and 10 samples per symbol
respectively.
1 2 4 8 10
10
-2
10
-1
10
0
No. of samples per bit (N)
BER
Figure 5: Effects of number of samples per symbol (N) on
BER when r=800 nm and f=0.01 bps.
400 nm 1 um 10 um 100 um
10
-2
10
-1
10
0
r (nm)
BER
Figure 6: Effects of communication range on BER when
N=10, f=0.01 bps.
0.01 0.02 0.05 0.1 0.5 1
10
-1.19
10
-1.16
10
-1.13
f (bps)
BER
Figure 7: Effects of transmission data rate on BER when
r=800 nm and N=10.
4 CONCLUSIONS
In this paper we have developed and evaluated the
performance of sampling-based optimum receiver
architecture of CEMC system. The proposed receiver
model should be valid for any type of input signal
transmission with any modulation format, e.g. pulse
amplitude modulation (PAM) transmission, and can
also be extended to detect signals with multilevel (M-
ary) amplitude modulation in CEMC system.
Bionanomachines existing in the nature can sense the
concentration of molecules at their receptors, which
may help implement sampling-based receivers
through engineering of bionanomachines. Finally, the
results presented in this paper will surely help a
molecular communication engineer to evaluate the
performance of a CEMC system in greater details.
REFERENCES
Akyildiz, I. F., Brunetti, F. and Blazquez, C., 2008.
"Nanonetworks: A New Communication Paradigm",
Computer Networks Journal (Elsevier), vol. 52, pp.
2260-2279.
Atakan, B. and Akan, O. B., 2010. "Deterministic capacity
of information flow in molecular nanonetworks",
Nano Communication Networks, vol. 1, no. 1, pp. 31-
42.
Berg, H. C., 1993. Random Walks in Biology, Princeton
University Press, NJ, USA. .
Bossert, W. H. and Wilson, E. O., 1963. "The analysis of
olfactory communication among animals", Journal of
theoretical biology, vol. 5, no. 3, pp. 443-469.
Haykin, S., 2000. Communication Systems, 4th edn, John
Wiley & Sons.
Kay, S. M., 1993. Fundamentals of statistical signal
processing, Vol. 2 Detection Theory, Englewood
Cliffs, NJ: PTR Prentice-Hall.
Mahfuz, M. U., Makrakis, D. and Mouftah, H. T. 2010a,
"Characterization of Molecular Communication
Channel for Nanoscale Networks", Proc.
BIOSIGNALS-2010, pp. 327, Spain, 20-23 January.
Mahfuz, M. U., Makrakis, D. and Mouftah, H. T. 2010b,
"On the characterization of binary concentration-
encoded molecular communication in nanonetworks",
Nano Communication Networks, vol. 1, no. 4, pp. 289-
300.
Moore, M.-., Suda, T. and Oiwa, K., 2009. "Molecular
Communication: Modeling Noise Effects on
Information Rate", NanoBioscience, IEEE
Transactions on, vol. 8, no. 2, pp. 169-180.
Nakano, T., Moore, M. J., Fang Wei, Vasilakos, A. V. and
Jianwei Shuai 2012. "Molecular Communication and
Networking: Opportunities and Challenges",
NanoBioscience, IEEE Transactions on, vol. 11, no. 2,
pp. 135-148.
BIOSIGNALS2013-InternationalConferenceonBio-inspiredSystemsandSignalProcessing
376