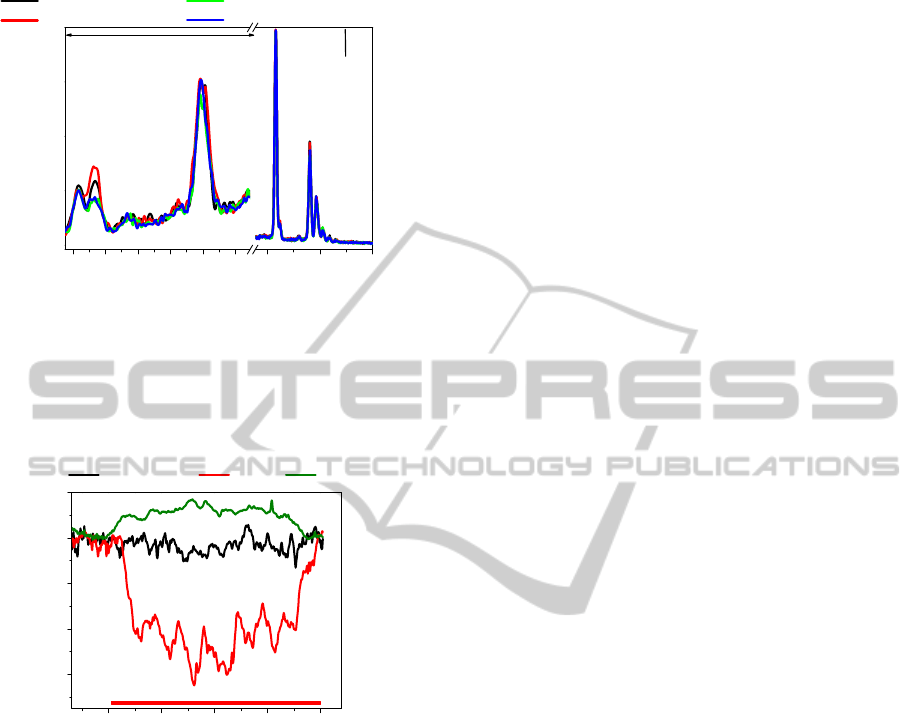
-100 -50 0 50 100
-300
-200
-100
0
100
spot 200 µm
Topography (nm)
X line (µm)
non illuminated 5 pulses 1 pulse
0,4 0,6 0,8 1,0 1,2 1,4 2 4 6
pu
se
centre of the crater
non illuminated
200 counts
Sb
Sb
Sb
Sb
Sb
S
S
intenzity x5
Ge
O
Sb
EDX signal
E
keV
pu
ses
centre of the crater
out of the crater
Figure 4: The EDX spectra of the samples, for location of
analysis see Fig. 1 and the text.
too small depth to expand the material by 50 nm.
Hence the heat should be dissipated deeper into the
bulk.
Figure 5: The line-scans of non-illuminated material,
material after 5 and single pulse (200 µm diameter of the
spot, pulse energy 0.2 mJ)
4 CONCLUSIONS
- The interaction of the 213 nm UV nanosecond
laser with chalcogenide glass (GeS
2
)
0.3
(Sb
2
S
3
)
0.7
has
been described;
- Influence of the most important parameters as
energy of the pulse, number of pulses and repetition
rate on the volume of the craters has been described;
- The shape of the craters is dependent on the
condition of illumination and at low energy pulses
created craters could be used for formation of
microlens array;
- Future work will be focused on the structural
changes of the material and analysis of the ablated
material.
ACKNOWLEDGEMENTS
The authors acknowledge financial support from the
project Grant Agency of the Czech Rep. (GPP108/
12/P044). We are indebted to Dr. M. Kincl for
technical assistance.
REFERENCES
Beadie, G., Rabinovich, W. S., Sanghera, J. & Aggarwal,
I. 1998. Fabrication of microlenses in bulk
chalcogenide glass. Opt. Commun., 152, 215-220.
Bryce, R. M., Nguyen, H. T., Nakeeran, et al. 2004. Direct
UV patterning of waveguide devices in As
2
Se
3
thin
films. J. Vac. Sci. Technol. A, 22, 1044-1047.
Fritze, M., Stern, M. B. & Wyatt, P. W. 1998. Laser-
fabricated glass microlens arrays. Opt. Let., 23, 141-
143.
Frumar, M., Frumarova, B., Nemec, P., Wagner, T.,
Jedelsky, J. & Hrdlicka, M. 2006. Thin chalcogenide
films prepared by pulsed laser deposition - new
amorphous materials applicable in optoelectronics and
chemical sensors. J. Non-Cryst. Solids, 352, 544-561.
Hitz, B., Ewing, J. & Hecht, J. 2012. Introduction to Laser
Technology, New York (USA), Wiley-IEEE Press.
Huang, Y., Liu, R., Lai, J. J. & Yi, X. J. 2008. Design and
fabrication of a negative microlens array. Opt. Laser
Technol., 40, 1047-1050.
Klapetek, P., Nečas, D. & Anderson, C. 2011. Gwyddion -
Free SPM data analysis software; v. 2.25.
Knotek, P., Arsova, D., Vateva, E. & Tichy, L. 2009.
Photo-expansion in Ge-As-S amorphous film
monitored by digital holographic microscopy and
atomic force microscopy. J. Optoelectron. Adv. M., 11,
391-394.
Knotek, P., Kincl, M., Tichy, L., Arsova, D., Ivanova, Z.
G. & Ticha, H. 2010. Oxygen assisted photoinduced
changes in Ge
39
Ga
2
S
59
amorphous thin film. J. Non-
Cryst. Solids, 356, 2850-2857.
Knotek, P. & Tichy, L. 2012 On photo-expansion and
microlens formation in (GeS
2
)
0.74
(Sb
2
S
3
)
0.26
chalcogenide glass. Mater. Res. Bull., 47, 4246-4251.
Knotek, P., Vlcek, M., Kincl, M. & Tichy, L. 2012. On the
ultraviolet light induced oxidation of amorphous As
2
S
3
film. Thin Solid Films, 520, 5472-5478.
Lim, C. S., Hong, M. H., Kumar, A. S., Rahman, M. &
Liu, X. D. 2006. Fabrication of concave micro lens
array using laser patterning and isotropic etching. Int.
J. Mach. Tool Manu., 46, 552-558.
Lin, C. G., Li, Z. B., Ying, L., Xu, Y. S., Zhang, P. Q.,
Dai, S. X., Xu, T. F. & Nie, Q. H. 2012. Network
Structure in GeS
2
-Sb
2
S
3
Chalcogenide Glasses: Raman
Spectroscopy and Phase Transformation Study. J.
Phys. Chem. C, 116, 5862-5867.
PHOTOPTICS2013-InternationalConferenceonPhotonics,OpticsandLaserTechnology
8