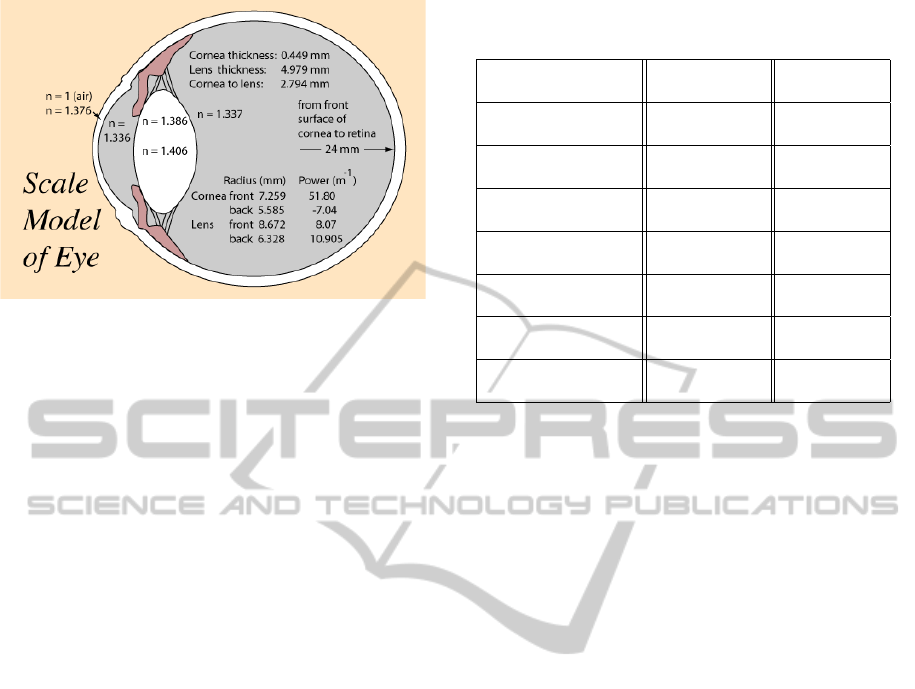
Figure 6: The refraction index of different eye organs, from
(eye, http://hyperphysics.phy-astr.gsu.edu/).
A real white object which is uniformly illumi-
nated can be considered as a superposition of plenty
of points. The corresponding calculation should be
done through integration. The object is considered to
be large, i.e. its angular size is much larger than the
human eye angular resolution, which is 4 minutes of
arc. A good example is a uniformly lit white wall.
Therefore the effects of straylight (Vos et al., 1976)
and dullness of the retinal focus are canceled. The
resulting retinal illuminance is the same as above.
Therefore an object illuminance of 1 klux results
in a retinal illuminance of only 15.1 lux for d = 6mm.
This means that the retinal illuminance is about 2 or-
ders of magnitude lower than the illuminance of the
observed object. Table 1 lists the calculated retinal il-
luminance values for various conditions. From the ta-
ble we read a retinal illuminance range between 0.034
mlux to 736.4 lux.
5 DISCUSSION
As we see from Fig. 4, the bulk substrate based photo-
sensor enters saturation in retinal illumances between
1 and 10 lux. Table 1 shows that this is already prob-
lematic for vision in living room and in street light.
For retinal illuminances below 1 lux, the photosensor
output voltage is not sensitive to illuminance changes,
so no contrast (for example edges) can be perceived
any more. The epitaxy based photosensor remains to
be linear in darker environments. However, because
of measurement setup limitations, we were unable to
verify its functioning for illuminances lower than 0.01
lux. Anyway, the necessity of using epitaxy based
photodiodes with low dark current is revealed.
Table 1: The calculated retinal illuminance in different con-
ditions, the observed object is white and 100% reflective.
Situation Environment Retinal
Illuminance Illuminance
Sun, summer, 70 klux 736.4 lux
d=5mm
Cloudy day, 2 klux 30.3 lux
d=6mm
Well lit office 1 klux 15.1 lux
d=6mm
Living room 120 lux 2.47 lux
d=7mm
Street light 16 lux 0.43 lux
d=8mm
Full moon 0.25 lux 8.5 mlux
d=9mm
Stars but no moon, 0.001 lux 0.034 mlux
clear night, d=9mm
6 CONCLUSIONS
We have fabricated photosensors containing photodi-
odes with an area of 15µm x 48µm on both a bulk and
an epitaxy substrate based CMOS process. Our mea-
surements showed that while the epitaxy based pho-
tosensor is linear in the range of 0.01 to 10 klux, the
photosensor fabricated on bulk substrate loses linear-
ity below 10 lux and enters saturation around 1 lux.
We developed a mathematical method to calculate the
retinal illuminance using optics and anatomy knowl-
edge. By comparing these results and the measured
photosensor characteristics, we discovered the advan-
tage of an epitaxy based process for photodiode fab-
rication in subretinal neural stimulator devices.
ACKNOWLEDGEMENTS
The authors would like to thank Dr. Walter G. Wrobel
and Mr. Johannes Groeger for their support on this
work.
REFERENCES
http://hyperphysics.phy-astr.gsu.edu/.
Allen, P. E. and Holberg, D. R. (1987). CMOS Analog Cir-
cuit Design. Oxford University Press.
Atchinson, D. A. and Smith, G. (2000). Optics of the human
eye. Butterworth-Heinemann Ltd.
Cinguino, P., Genova, F., Rigo, C., and Stano, A. (1985).
Low dark current ingaas pin photodiodes grown
by molecular beam epitaxy. Electronics Letters,
21(4):139 –140.
AnalysisofPhotosensorPropertiesforVisualNeuralStimulators
43