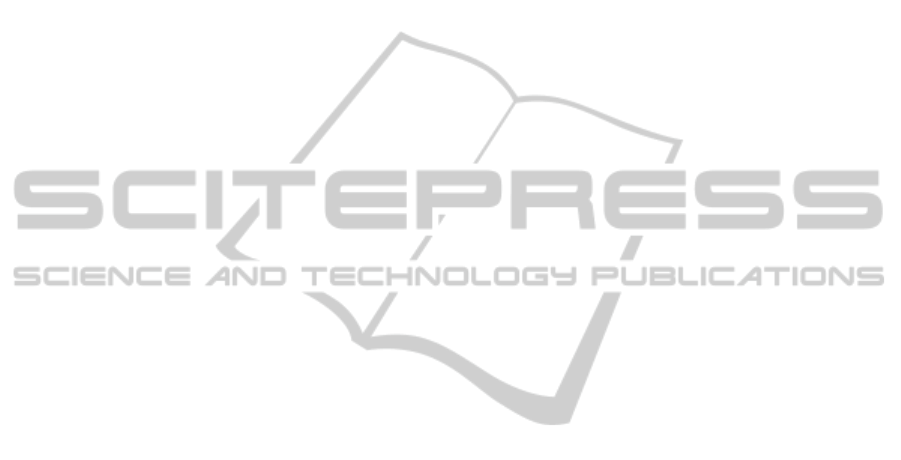
fuels fundamental studies when new questions or
scientific obstacles have been highlighted by pre-
clinical or clinical trials. Through the example of
vocal cord fluorescence imaging, this position paper
illustrates that the concept of bi-directional
translational research should be applied to all work
aiming to develop new medical devices.
ACKNOWLEDGEMENTS
The studies presented in this paper are conducted in
the frame of the STREP project "µRALP" funded by
the European Commission's 7th Framework
Program. It addresses the FP7-ICT-2011-7 call for
cognitive and robotic systems.
REFERENCES
Adbat, F., Amouroux, M., et al., 2012, Hybrid feature
selection and SVM-based classification for mouse skin
precancerous stages diagnosis from bimodal
spectroscopy, Opt. Express, Vol. 20, pp. 228-244.
Arens, C., Reussner, D., et al., 2007, Indirect fluorescence
laryngoscopy in the diagnosis of precancerous and
cancerous laryngeal lesions, Eur. Arch. Oto-Rhino-L.,
Vol. 264, pp. 621–626.
Diaz-Ayil, G., Amouroux, M., et al., 2007, Bimodal
spectroscopic evaluation of ultra violet-irradiated
mouse skin inflammatory and precancerous stages:
instrumentation, spectral feature extraction/selection
and classification (k-NN, LDA and SVM), Eur. Phys.
J-Appl. Phys. Vol. 47, pp. 12707.
Gillenwater, A., Jacob, R., et al., 1998, Noninvasive
Diagnosis of Oral Neoplasia Based on Fluorescence
Spectroscopy and Native Tissue Autofluorescence,
Arch. Otolaryngol., Vol.124, pp. 1251-1258.
Kraft, M., Betz, C. S., et al., 2010, Value of fluorescence
endoscopy for the early diagnosis of laryngeal cancer
and its precursor lesions, Head Neck, Vol. 33
pp. 941-948.
Lane, P.M., Gilhuly, T., et al., 2006, Simple device for the
direct visualization of oral-cavity tissue fluorescence,
J. Biomed. Opt., Vol 11, pp. 024006-1 – 024006-7.
Mehrotra, R., Singh, M., et al., 2010, A cross-sectional
study evaluating chemiluminescence and
autofluorescence in the detection of clinically
innocuous precancerous and cancerous oral lesions, J.
Am. Dent. Assoc., Vol. 141, pp. 151–156.
Muller, M. G., Georgakoudi, I., et al., 2001, Intrinsic
fluorescence spectroscopy in turbid media
disentangling effect of scattering and absorption, Appl.
Optics, Vol. 40, pp. 4633-4646.
Patton, L. L., Epstein, J. B., et al., 2008, Techniques for
oral cancer examination and lesion diagnosis: a
systematic review of the literature, J. of American
Dental Association JADA, Vol. 139, pp. 896-905.
Piazza, C., Bon, F. D., et al., 2011, Biologic endoscopy’:
optimization of upper aerodigestive tract cancer
evaluation, Otolaryng. Head Neck, Vol. 19, pp. 67–76.
Poh, C. F., Zhang, L. et al., 2006, Fluorescence
visualization detection of field alterations in tumor
margins of oral cancer patients, Clin. Cancer Res.,
Vol. 12, pp.6716-6722.
Rethman, M. P., Carpenter, W., et al., 2010, Evidence-
Based Clinical Recommendations Regarding
Screening for Oral Squamous Cell Carcinomas, J. of
American Dental Association JADA, Vol. 141, pp.
509-520.
Richards-Kortum, R; Sevick-Muraca, E., 1996,
Quantitative optical spectroscopy for tissue diagnosis,
Annu. Rev. Phys. Chem. Vol. 47, pp. 555–606.
Shin, D., Vigneswaren, N. et al., 2010, Advances in
fluorescence imaging techniques to detect oral cancer
and its precursors, Future Oncol., Vol. 6, pp. 1143–
1154.
Wagnieres, G. A., Star, W. M., et al., 1998, In vivo
fluorescence spectroscopy and imaging for
oncological applications, Photochem. Photobiol.,
Vol.68, pp.603–632.
Wu, J., Feld, M. S., et al., 1993, Analytical model for
extracting intrinsic fluorescence in turbid media, Appl.
Optics, Vol. 32, pp. 3585-3595.
ReverseTranslationalResearch-HowClinicalTrialsonFluorescenceImagingforVocalCordCancerFuelsFundamental
Research
287