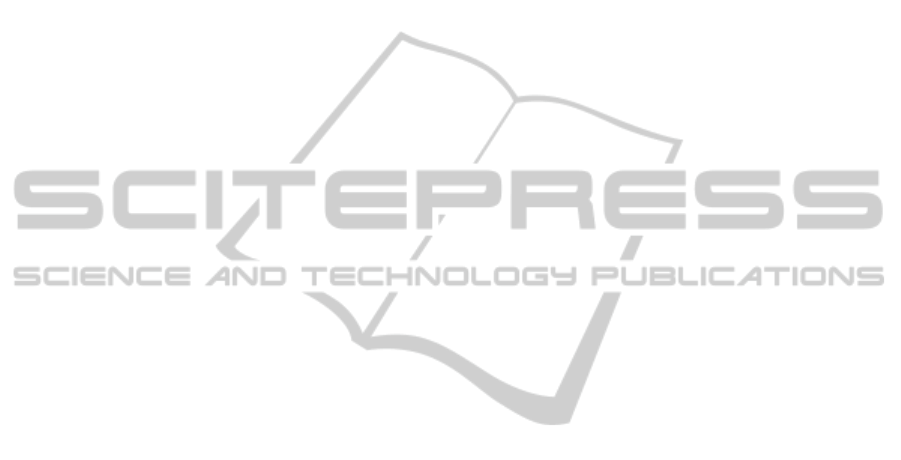
4 DISCUSSION OF RESULTS
Experimental data for growth factor release seem to
indicate that using electric pulse stimulation in the
nanosecond range for platelet activation would result
in a different growth factor profile compared to
bovine thrombin. For example, existing data in
literature show that typical physiological platelet
activators – thrombin, ADP, collagen – tend to have
relatively similar growth release profiles for VEGF
(Maloney, 1998). While the use of nsPEF can offer
the advantage of platelet activation without the use
animal based activators already on the market –
bovine thrombin, bovine derived collagen – one
would have to establish the in vivo wound healing
effects of this different growth factor mix.
There are additional questions to be answered
with in vitro experiments before considering a
clinical path, such as the effects of nsPEF on other
components in the platelet rich plasma (white blood
cells - WBC, red blood cells - RBC). Additionally,
one would need to understand how nsPEF act on
platelet rich plasma compositions produced by the
numerous platelet separation machines
commercially available.
Different devices produce various versions of
platelet rich plasmas – RBC count, WBC count,
platelet enrichment, viability of PRP components
can vary. These versions of PRP may not only be
different from a biology point of view, but also they
could exhibit different electrical behaviours, which
may need to be accounted when one would design a
commercial instrument. Finally, experiments
described in this work use typical electroporation
cuvettes that may need additional qualification for
any human in vivo work.
5 CONCLUSIONS
The use of nanosecond electric field pulses for ex-
vivo platelet activation is an exciting novel
technology, which opens promising opportunities for
a truly autologous solution in the platelet gel space,
by accomplishing platelet aggregation and growth
factor release without using animal derived
activators. It should be noted that previous
researchers demonstrated various means for platelet
activation that do not include the use of thrombin or
other bio-chemical vectors – based on the use of
physical means such as ultrasound (Poliachik, 2001),
light (Verhaar, 2008) and high speed centrifugation
(Mazzucco, 2009). However the use of nsPEF for
platelet activation has significant advantages over
previous attempts to bypass the use of biochemical
activators: speed (~ 1 s exposure to electric field
pulses), process control, low cost, simplified
workflow. The wide potential applicability of
platelet gel therapy – healing of non-healing wounds
such as diabetic foot ulcers, haemostasis, and
reduction of wound infection – may be further
fostered by the introduction of this rapid, low cost,
easy access, truly autologous, non-animal derived
platelet activation method.
ACKNOWLEDGEMENTS
The authors of this paper would like to thank
Barbara Hargrave, Richard Heller (Old Dominion
University) and Reginald Smith (GE Global
Research) for valuable discussions and suggestions
throughout this research. The authors thank Yeong-
Jer Chen (Old Dominion University) for building
and providing technical assistance with the
nanosecond pulse generator used for experiments
presented here.
REFERENCES
Tate, K. S. and Crane, D. M., 2010. Platelet rich plasma
grafts in musculoskeletal medicine. In Journal of
Prolotherapy, Volume 2, Issue 2.
Driver, V. R., Hanft, J., Fylling, C. P., Beriou, J. M., 2006.
A prospective, randomized, controlled trial of
autologous platelet-rich plasma gel for the treatment of
diabetic foot ulcers. In Ostomy/Wound Management,
52(6).
Lacci, K. M. and Dardik, A., 2010. Platelet-rich plasma :
support for its use in wound healing. In Yale Journal
of biology and medicine, 83.
Gunaydin, S., McCusker, K., Sari, T., Onur, M., Gurpinar,
A., Sevim, H., Atasoy, P., Yorgancioglu, C., Zorlutuna
Y., 2008. Clinical impact and biomaterial evaluation
of autologous platelet gel in cardiac surgery. In
Perfusion, 23, 179-186.
Alexander, W., 2009. Meeting highlights of the 30th
American College of Clinical Pharmacy annual
meeting. In Pharmacy & Therapeutics, vol. 34, no. 12.
Cada, D. J., Levien, T. Baker, D. E., 2008. Thrombin,
Topical (Recombinant). In Hospital Pharmacy
Volume 43, Number 7, Wolters Kluwer Health, Inc.
Beebe, S. J. and Schoenbach, K. H., 2005. Nanosecond-
pulsed electric fields: a new stimulus to activate
intracellular signalling. In Journal of Biomedicine and
Biotechnology. 4:297-300.
Zhang, J., Blackmore, P. F., Hargrave, B. Y., Xiao, S.,
Beebe, S.J, Schoenbach, K.H., 2008. Nanosecond
Ex-vivo Platelet Activation using Electric Pulse Stimulation
207