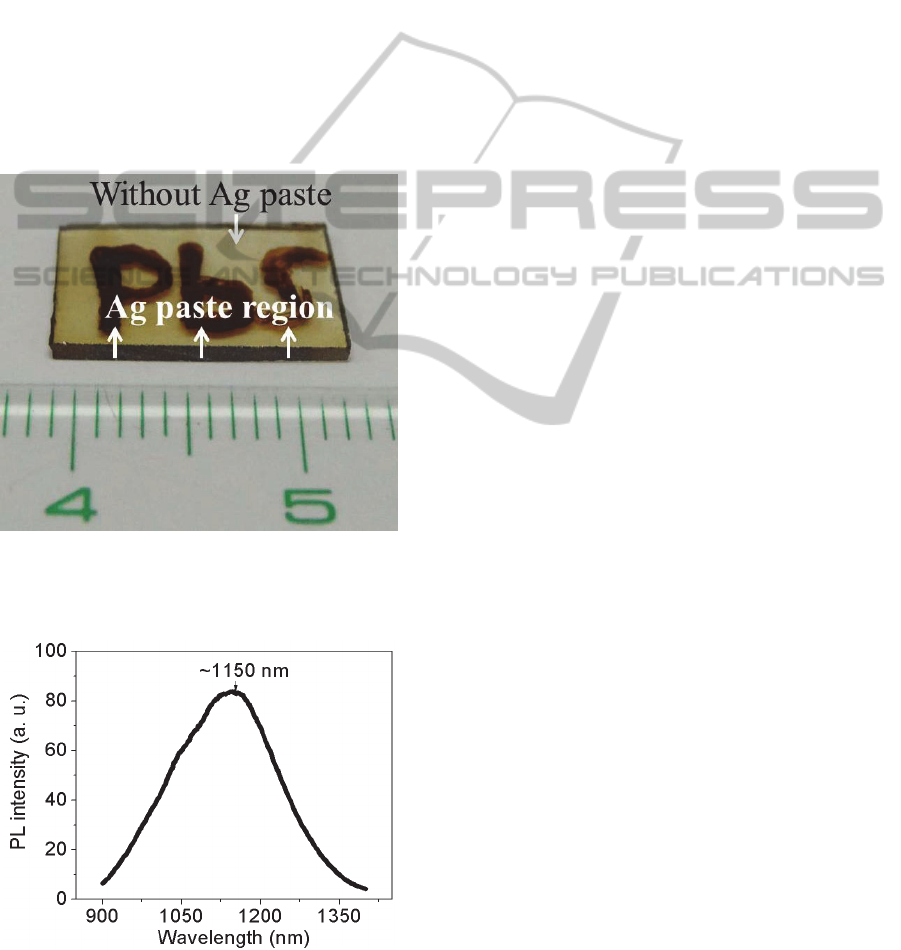
formed and were confirmed by TEM image. PbS
QDs in Ag
+
-migrated glass surface photoluminesced
the longer wavelengths than those in Ag
+
-free glass
when heat-treatment temperatures were 440 and
450°C. PbS QDs can also precipitate at temperatures
as low as 420 or 430°C after Ag
+
ion migration. Ag
concentration analyzed by EDX indicated that PbS
QDs could precipitate within ~30 μm layer from the
glass surface. XPS and optical absorption spectra
confirmed that Ag NPs formed at the initial stage of
heat treatment. Ag NPs thus formed provided the
nucleating sites and promote the formation of PbS
QDs in glasses. Solid-state Ag
+
ion migration
method can effectively control the spatial
distribution of PbS QDs in glasses, and it has the
potentials on space-selective formation of PbS QDs
in glasses for micro- or nano-photonic devices.
Figure 7: Photograph of PbS QDs with “PbS” word in
glass. Glass was subjected to Ag
+
ion migration at 320°C
for 2 h and then heat treatment at 420°C for 10 h.
Figure 8: PL spectrum from “PbS” word in Figure 7. Glass
was subjected to Ag
+
ion migration at 320°C for 2 h and
then heat treatment at 420°C for 10 h.
ACKNOWLEDGEMENTS
This work was supported by Basic Science Research
(2010-0022407), Priority Research Centers (2012-
046983) and World Class University (R31-30005)
Programs through the National Research Foundation
of Korea funded by the Ministry of Education,
Science and Technology.
REFERENCES
Borrelli, N. F. and Smith, D. W. (1994). Quantum
Confinement of PbS Microcrystals in Glass. J. Non-
Cryst. Solids, 180, 25-31.
Heo, J. and Liu, C. (2007). PbS Quantum-Dots in Glass
Matrix for Universal Fiber-Optic Amplifier. J. Mater.
Sci: Mater Electron., 18, S135-39.
Lamaestre, R. E., Majimel, J., Jomard, F. and Bernas, H.
(2005). Synthesis of Lead Chalcogenide Nanocrystals
by Sequential Ion Implantation in Silica. J. Phys.
Chem. B, 109, 19148-55.
Liu, C., Kwon, Y. K., Heo, J., Kim, B. H. and Sohn, I.
(2010). Controlled Precipitation of Lead Sulfide
Quantum Dots in Glasses Using the Femtosecond
Laser Pulses. J. Am. Ceram. Soc., 93, 1221-24.
Malyarevich, A. M., Yumashev, K. V. and Lipovskii, A.
A. (2008). Semiconductor-Doped Glass Saturable
Absorbers for Near-Infrared Solid-State Lasers. J.
Appl. Phys., 103, 081301- 25.
Najafi, S. I. (1992). Introduction to Glass Integrated
Optics. Artech House, Boston.
Stookey, S. D. (1959). Catalyzed Crystallization of Glass
in Theory and Practice. Ind. Eng. Chem., 51, 805-08.
Wang, P. W. (1997). Formation of Silver Colloids in
Silver Ion-exchanged Soda-lime Glasses during
Annealing. Appl. Surf. Sci., 120, 291-98.
Wise, F. W. (2000). Lead Salt Quantum Dots: the Limit of
Strong Quantum Confinement. Acc. Chem. Res., 33,
773-80.
Woggon, U., (1997). Optical Properties of Semiconductor
Quantum Dots. Springer, Berlin.
Xu, K., Liu, C., Dai, S., Shen, X., Wang, X. and Heo, J.
(2011). Influence of Silver Nanoclusters on Formation
of PbS Quantum Dots in Glasses. J. Non-Cryst. Solids,
357, 2428-30.
Xu, K. and Heo, J. (2012a). Lead Sulfide Quantum Dots in
Glasses Controlled by Silver Diffusion. J. Non-Cryst.
Solids, 358, 921-24.
Xu, K. and Heo, J. (2012b). Effect of Silver Ion-Exchange
on the Precipitation of Lead Sulfide Quantum Dots in
Glasses. J. Am. Ceram. Soc.,95, 2880-84.
Yong, K. T., Sahoo, Y., Choudhury, K. R., Swihart, M. T.,
Minter, J. R. and Prasad, P. N. (2006). Control of the
Morphology and Size of PbS Nanowires Using Gold
Nanoparticles. Chem. Mater., 18, 5965-72.
Solid-stateAg+IonMigrationfortheControlledPrecipitationofPbSQuantumDotsinGlasses
87