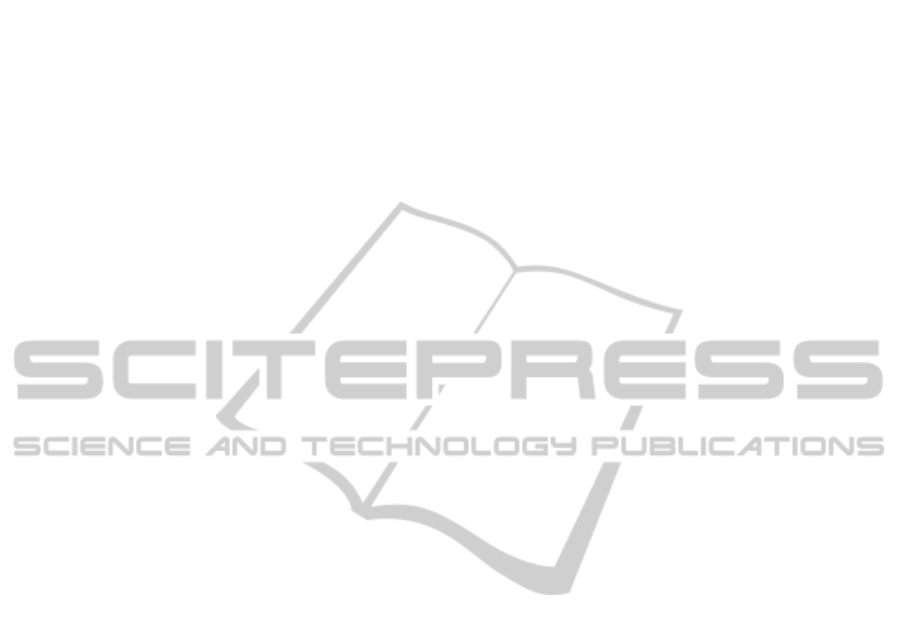
vol. QE-10, no. 4, pp. 450-457.
Lahoz, F., Shepherd, D. P., Wilkinson, J. S. & Hassan, M.
A., 2008. Efficient blue upconversion emission due to
confined radiative energy transfer in Tm
3+
–Nd
3+
co-
doped Ta
2
O
5
waveguides under infrared-laser
excitation. Opt. Commun., vol. 281, pp. 3691-3694.
Lee, D. J., Heo, J. & Park, S. H., 2003. Energy transfer
and 1.48 μm emission properties in chalcohalide
glasses doped with Tm
3+
and Tb
3+
. J. Non-Cryst.
Solids, vol. 331, pp. 181-189.
Lucas, J. et al., 1978. Preparation and optical properties of
neodymium fluorozirconate glasses. J. Non-Cryst.
Solids, vol. 27, pp. 273-283.
Lu, K. & Dutta, N. K., 2001. Spectroscopic properties of
Nd-doped glass for 944 nm laser emission. J. Appl.
Phys., vol. 89, no. 6, pp. 3079-3083.
Martinez, A., Zenteno, L. A. & Kuo, J. C. K., 1998.
Optical and spectroscopic characterization of Nd-
doped aluminosilicate fiber preforms made by the
MCVD method using chelate delivery. Appl. Phys. B,
vol. 67, pp. 17-21.
Milam, D. & Weber, M. J., 1976. Measurement of
nonlinear refractive-index coefficients using time-
resolved interferometry: application to optical
materials for high-power neodymium lasers. J. Appl.
Phys., vol. 47, pp. 2497-2501.
Peterka, P. et al., 2004. Theoretical modelling of S-band
thulium-doped silica fibre amplifiers. Opt. Quant.
Electron., vol. 36, pp. 201-212.
Quimby, R. S. & Miniscalco, W. J., 1989. Continuous-
wave lasing on a self-terminating transition. Appl.
Opt., vol. 28, no. 1, pp. 14-16.
Rakov, N., Gómez, L. A., Rátiva, D. J. & Maciel, G. S.,
2002. Blue upconversion enhancement by a factor of
200 in Tm
3+
-doped tellurite glass by codoping with
Nd
3+
ions. J. Appl. Phys., vol. 92, no. 10, pp. 6337-
6339.
Rakov, N., Gómez, L. A., Rátiva, D. J. & Maciel, G. S.,
2009. Blue upconversion emission from Tm
3+
sensitized by Nd
3+
in aluminum oxide crystalline
ceramic powders. Appl. Phys. B, vol. 94, pp. 199-202.
Shen, S. et al., 2002. Tellurite glasses for broadband
amplifiers and integrated optics. J. Am. Ceram. Soc.,
vol. 85, no. 6, pp. 1391-1395.
Stokowski, S. E., Saroyan, R. A. & Weber, M. J., 1981.
Nd-doped laser glass spectroscopic and physical
properties. Lawrence Livermore National Laboratory,
M-095, Rev. 2, 1.
Tanabe, S., 2002. Rare-earth-doped glasses for fiber
amplifiers in broadband telecommunication. C. R.
Chim., vol. 5, no. 5, pp. 815-824.
Tanabe, S., Feng, X. & Hanada, T., 2000. Improved
emission of Tm
3+
doped glass for a 1.4-μm amplifier
by radiative energy transfer between Tm
3+
and Nd
3+
.
Opt. Lett., vol. 25, no. 11, pp. 817-819.
Thomas, I. M., Payne, S. A. & Wilke, G. D., 1992. Optical
properties and laser demonstrations of Nd-doped sol-
gel silica glasses. J. Non-Cryst. Solids, vol. 151, no. 3,
pp. 183-194.
Walsh, B. M. & Barnes, N. P., 2004. Comparison of
Tm:ZBLAN and Tm:silica fiber lasers; spectroscopy
and tunable pulsed laser operation around 1.9 μm.
Appl. Phys. B, vol. 78, pp. 325-333.
Watekar, P. R., Ju, S., Boo, S. & Han, W.-T., 2005. Linear
and non-linear optical properties of Yb
3+
/Tm
3+
co-
doped alumino-silicate glass prepared by sol-gel
method. J. Non-Cryst. Solids, vol. 351, pp. 2446-2452.
Watekar, P. R., Ju, S. & Han, W.-T., 2006. A small-signal
power model for Tm-doped silica-glass optical fiber
amplifier. IEEE Photon. Technol. Lett., vol. 18, no.
19, pp. 2035-2037.
Weber, M. J., Lynch, J. E., Blackburn, D. H. & Cronin, D.
J., 1983. Dependence of the stimulated emission cross
section of Yb
3+
on host glass composition. IEEE J.
Quantum Electron., vol. 19, pp. 1600-1608.
Yanbo, Q. et al., 2006. Spectroscopic properties of Nd
3+
-
doped high silica glass prepared by sintering porous
glass. J. Rare Earth., vol. 24, pp. 765-770.
Yang, Z., Luo, L. & Chen, W., 2006. The 1.23 and 1.47
μm emissions from Tm
3+
in chalcogenide glasses. J.
Appl. Phys., vol. 99, no. 7, pp. 076107-3.
Zabicky, J. (ed.), 2009. The chemistry of metal enolates,
part 1. John Wiley & Sons Ltd.
Zhang, J., Chung, W. J., Zhao, X. & Heo, J., 2010. Nd
3+
sensitized blue upconversion luminescence in
Nd
3+
/Pr
3+
co-doped Ge–Ga–S–CsBr chalcohalide
glasses. J. Non-Cryst. Solids, vol. 356, pp. 2406-2408.
Zhang, J. W. et al., 2006. Optical amplification in Nd
3+
doped electro-optic lanthanum lead zirconate titanate
ceramics. Appl. Phys. Lett., vol. 89, pp. 061113.
Zhou, B., Lin, H. & Pun, E.Y.-B., 2010. Tm
3+
-doped
tellurite glasses for fiber amplifiers in broadband
optical communication at 1.20 µm wavelength region.
Opt. Express, vol. 18, no.18, pp. 18805-18810.
Zou, X. & Toratani, H., 1996. Spectroscopic properties
and energy transfers in Tm
3+
singly- and Tm
3+
/Ho
3+
doubly-doped glasses. J. Non-Cryst. Solids, vol. 195,
pp. 113-124.
PHOTOPTICS2013-InternationalConferenceonPhotonics,OpticsandLaserTechnology
32