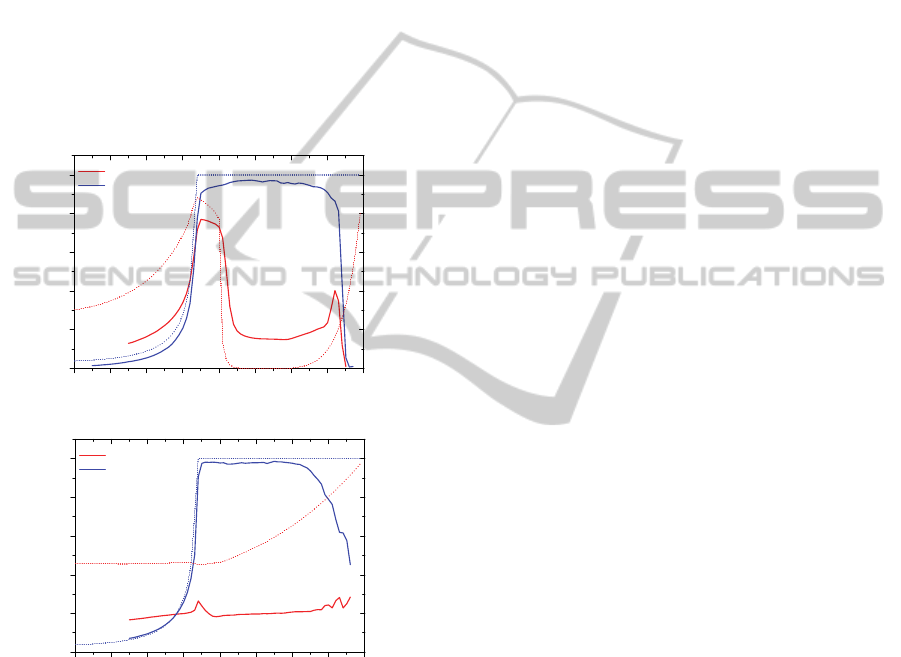
predicted by simulation which is also plotted in the
same figure with dotted line. However, the residual
specular reflection at incidence angles larger than
55° does not go to zero. The reason is considered to
be due to imperfection of the PCM grating.
Next, to conduct the measurement for a
crystalline GST grating, the as-deposited amorphous
GST grating sample was annealed at 200°C for 5
minutes to convert into the crystalline state. The
measured results are shown in Fig. 4(b). As
expected, the specular reflection does not show a
fast drop. However, the overall amplitude is quite
lower than simulation which might also be due to
fabrication error and large absorption in crystalline
GST. Especially the thickness of GST was suspected
to be thicker than designed.
10 20 30 40 50 60 70 80 90
0.0
0.2
0.4
0.6
0.8
1.0
Specular reflectivity [a.u.]
Incidence angle [
o
]
grating area
no grating area
(a) Amorphous GST grating
10 20 30 40 50 60 70 80 90
0.0
0.2
0.4
0.6
0.8
1.0
Specular reflectivity [a.u.]
Incidence angle [
o
]
grating area
no grating area
(b) Crystalline GST grating
Figure 4: Measured specular reflection efficiency of
s-polarized light for a GST grating. The optical
wavelength is set as 1.55 μm. The specular reflection of
no-grating area is also measured as a reference. In
addition, the simulation results are plotted in dotted lines.
5 CONCLUSIONS
To verify the concept of a spatial grating-type PCM
optical switch, we have fabricated the designed
grating structure using electron-beam lithography
and laser interference lithography techniques. The
laser interference lithography is especially useful to
generate large area periodical pattern. The static
switching characteristic of the grating was verified
by optical diffraction measurements for both the
amorphous and crystalline states, and they agree
roughly with the theoretical expectations. We
believe that further precise control in fabrication will
improve the experimental results. In the future,
dynamic phase change by laser pulse will be
necessary to testify the switching actions. The
optical switch can be driven by a more absorptive
visible wavelength to switch between its two phase
states, just as what have been done in an optical disk
system. In addition, PCM with a lower absorption at
1.55 m is greatly desirable in order to improve the
switching efficiency of the crystalline state switch.
ACKNOWLEDGEMENTS
This study was supported by a grant from the
Industrial Technology Research Program, 2011, of
the New Energy and Industrial Technology
Development Organization (NEDO), Japan. A part
of the fabrication work was conducted at AIST
Nano-Processing Facility, supported by
“Nanotechnology Network Japan” of the Ministry of
Education, Culture, Sports, Science and Technology
(MEXT), Japan.
REFERENCES
Burr, G. W. et al., 2010. Phase change memory
technology. Journal of Vacuum Science & Technology
B: Microelectronics and Nanometer Structures, 28(2),
pp.223–262. doi:10.1116/1.3301579.
Fucetola, C. P., Korre, H. & Berggren, K.K., 2009. Low-
cost interference lithography. Journal of Vacuum
Science & Technology B: Microelectronics and
Nanometer Structures, 27(6), p.2958. doi:10.1116/
1.3245990.
Ikuma, Y., Saiki, T. & Tsuda, H., 2008. Proposal of a
small self-holding 2×2 optical switch using phase-
change material. IEICE Electronics Express, 5(12),
pp.442–445. doi:10.1587/elex.5.442.
Ikuma, Y. et al., 2010. Small-sized optical gate switch
using Ge
2
Sb
2
Te
5
phase-change material integrated
with silicon waveguide. Electronics Letters, 46(5),
pp.368–369. doi:10.1049/el.2010.3588.
Li, L., 1997. New formulation of the Fourier modal
method for crossed surface-relief gratings. Journal of
the Optical Society of America A, 14(10), pp.2758–
2767. doi:10.1364/JOSAA.14.002758.
Moharam, M. G. & Gaylord, T. K., 1981. Rigorous
coupled-wave analysis of planar-grating diffraction.
PHOTOPTICS2013-InternationalConferenceonPhotonics,OpticsandLaserTechnology
104