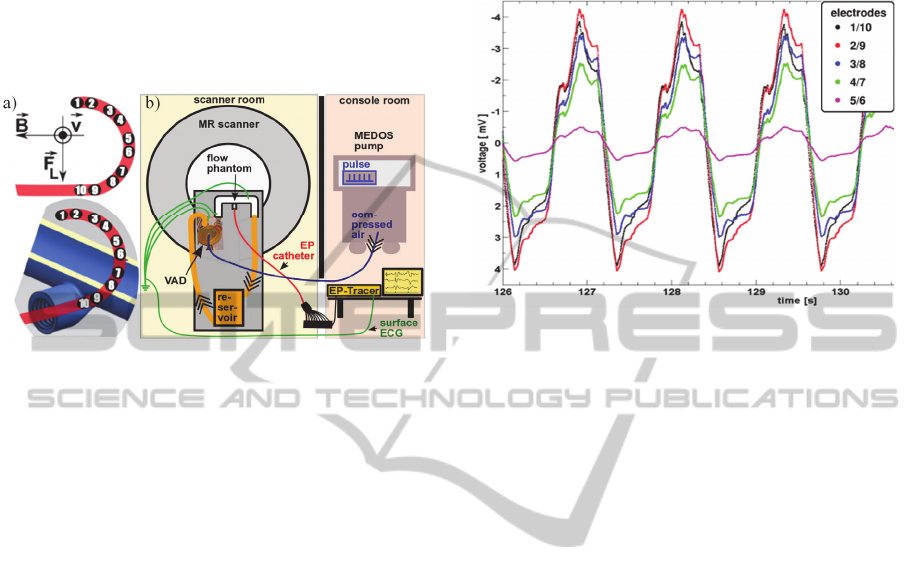
between the electrodes 2/9. However, this deviation
is within the limits of the experimental accuracy. A
linear decrease of the potential with a decrease in d
is observed (not shown). Additionally, it is important
to note the underlying unknown shift and scaling of
the voltage signal in the vertical direction. This is
due to the impact of the high pass filter of the pre-
amplifier on the MHD signal.
Figure 1: EP catheter positioning in the phantom and its
orientation with respect to the flow velocity v, the external
magnetic field B, and the Lorentz force F
L
(a), and
experimental setup (b).
4 DISCUSSION
The investigation of MHD potentials detected with
common EP equipment is important for developing
EP exam procedures in a MR environment.
Therefore, a MRI compatible flow circuit was
successfully established. The linear dependency
between the measured potential and d (as well as B
0
,
both not shown) predicted by theory was clearly
observed and validates the model system. Typical
electrode distances as between the electrodes 5/6
revealed a significant MHD potential which cannot
be neglected. The non-conductive walls of the flow
phantom are not expected to bias the outcome of the
measurement significantly since studies revealed
that vessel wall conductivity may be neglected
(Abdallah et al., 2008). Further investigations will
analyse the impact of different recording modalities
such as hardware filters on the detection of the MHD
signal. Furthermore, glycerol will be added to the
saltwater for simulating the viscosity and the density
of blood.
The time course of MR velocity data at the
catheter position (not shown) agrees very well with
the EP-Tracer data (apart from filter effects). Hence,
additional simple and quick MR flow measurements
at the location of interest during an EP exam may be
used to remove the MHD related potential from
intracardiac ECG signals representing an essential
step towards diagnostically valuable data.
Additionally, in vivo data, e.g. from animal models,
is required for the validation of these methods.
Figure 2: MHD potential time course.
ACKNOWLEDGEMENTS
EUROSTARS Program Grant #01QE1004D.
REFERENCES
Abdallah, D. A., Drochon, A., Robin, V., Fokapu, O.,
2008. Magnetohydrodynamic flow of blood: Influence
of the simplifying assumptions in calculations. J. of
Biomechanics 41 (S1), S269.
Gupta, A., Weeks, A. R., Richie, S. M., 2008. Simulation
of elevated T-waves of an ECG inside a static
magnetic field (MRI). IEEE transactions on
biomedical engineering 55 (7), 1890-96.
Josephson, M. E., 2008. Clinical cardiac electro-
physiology techniques and interpretations, Lippincott
Williams & Wilkins. Philadelphia, 4
th
edition.
Schneider, Ch., 2005. Das EPU-Labor, Steinkopff Verlag.
Würzburg.
Tenforde, T. S., Gaffey, C. T., Moyer, B. R., Budinger, T.
F., 1983. Cardiovascular alterations in Macaca
monkeys exposed to stationary magnetic fields:
Experimental observations and theoretical analysis.
Bioelectromagnetics 4, 1-9.
Tenforde, T. S., 2005. Magnetically induced electric fields
and currents in the circulatory system. Progress in
Biophysics & Molecular Biology 87, 279-288.
Tse, Z. T. H., Dumoulin, C. L., Watkins, R., Byrd, I.,
Schweitzer, J., Kwong, R. Y., Michaud, G. F.,
Stevenson, W. G., Schmidt, E. J., 2012. MRI-
compatible voltage-based electro-anatomic mapping
system for cardiac electrophysiological interventions.
In 20
th
ISMRM, oral presentation #206.