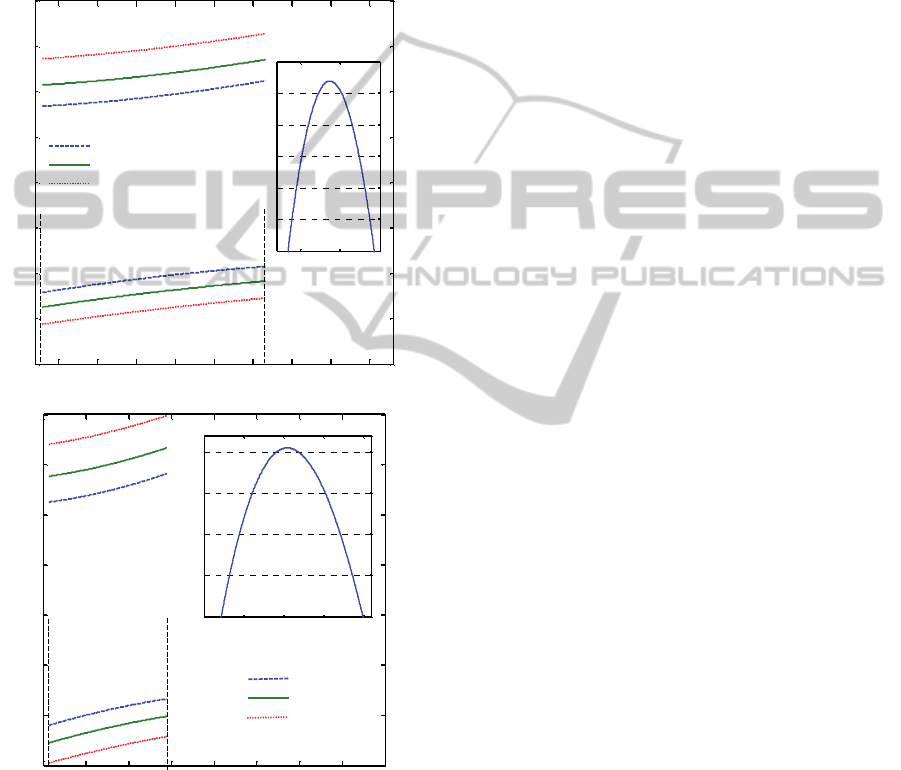
nm). Their variation in this region reveals the effect
of nonlinear coefficient and peak power significantly.
When P increases from 10000 to 40000 m
-1
the
signal and idler wavelengths shift away from each
other. The phase-matching curves observed for
HMOF with two ZDW is as shown in Fig. 4(b). In
Fig. 4(b) the similar phase-matching curves were
observed between the first and second ZDW (1241
and 1269 nm) for different P values.
Figure 4: Phase-matching curves for (a) Step Index fiber
and (b) Hybrid microstructured optical fiber.
4 CONCLUSIONS
The HMOF has the bandwidth of 104 nm and it has
been shown that for the given set of nonlinearity
coefficient and peak power a parametric gain 33 dB
for step index fiber and 29 dB for HMOF can be
obtained. While, the phase-matching curves for the
step index and HMOF with two ZDW were
observed to be between their respective ZDWs. It is
concluded that for broad bandwidth it is very much
necessary to have very small values of
2
and
4
simultaneously.
ACKNOWLEDGEMENTS
This research was by the Ministry of Education, Culture,
Sports, Science and Technology under the support
Program for Forming Strategic Research Infrastructure
(2011-2015).
REFERENCES
Agrawal, G. 2000a. Nonlinear Fiber Optics. Nonlinear
Science At The Dawn Of The 21st Century, 195-211.
Agrawal, G. P. 2000b. Nonlinear Fiber Optics, Springer.
Chaudhari, C., Liao, M., Suzuki, T. & Ohishi, Y. 2012.
Chalcogenide Core Tellurite Cladding Composite
Microstructured Fiber For Nonlinear Applications.
Journal Of Lightwave Technology, 30, 2069-2076.
Djordjevic, I. B. 2011. Deep-Space And Near-Earth
Optical Communications By Coded Orbital Angular
Momentum (Oam) Modulation. Opt. Express, 19,
14277-14289.
Droques, M., Barviau, B., Kudlinski, A., Bouwmans, G. &
Mussot, A. Year. Simple Method For Measuring The
Zero-Dispersion Wavelength In Optical Fibers. In:
The European Conference On Lasers And Electro-
Optics, 2011. Optical Society Of America.
Gao, W., Liao, M., Cheng, T., Suzuki, T. & Ohishi, Y.
2013. Tunable Brillouin-Erbium Comb Fiber Laser In
A Linear Cavity With A Single-Mode Tellurite Fiber.
Ghosh, G. & Yajima, H. 1998. Pressure-Dependent
Sellmeier Coefficients And Material Dispersions For
Silica Fiber Glass. Journal Of Lightwave Technology,
16, 2002.
Lavoute, L., Knight, J. C., Dupriez, P. & Wadsworth, W.
J. 2010. High Power Red And Near-Ir Generation
Using Four Wave Mixing In All Integrated Fibre
Laser Systems. Optics Express, 18, 16193-16205.
Mussot, A., Lantz, E., Durecu-Legrand, A., Simonneau,
C., Bayart, D., Sylvestre, T. & Maillotte, H. 2006.
Zero-Dispersion Wavelength Mapping In Short
Single-Mode Optical Fibers Using Parametric
Amplification. Photonics Technology Letters, Ieee, 18,
22-24.
Nugent, P. W., Shaw, J. A. & Piazzolla, S. 2009. Infrared
Cloud Imaging In Support Of Earth-Space Optical
Communication. Opt. Express, 17, 7862-7872.
Parolari, P., Marazzi, L., Rognoni, E. & Martinelli, M.
2005. Influence Of Pump Parameters On Two-Pump
1225 1230 1235 1240 1245 1250 1255 1260 1265
1050
1100
1150
1200
1250
1300
1350
1400
1450
Pump Wavelength (nm)
Signal/Idler Wavelength (nm)
SI (D=0.944
m)
1200 1250 1300
-5
-4
-3
-2
-1
0
1
Wavelength (nm)
Dispersion (ps/km-nm)
P=10000 m
-1
P=20000 m
-1
P=40000 m
-1
1240 1250 1260 1270 1280 1290 1300 1310 1320
1100
1150
1200
1250
1300
1350
1400
1450
Pum
Wavelen
th
nm
S ig n a l/Id le r W a v e le n g th (n m )
MOF (D=0.9
m)
1150 1200 1250 1300 1350
-10
-7.5
-5
-2.5
0
Wavelength (nm)
Dispersion (ps/km-nm)
P=10000 m
-1
P=20000 m
-1
P=40000 m
-1
a
b
OPTICS2013-InternationalConferenceonOpticalCommunicationSystems
410