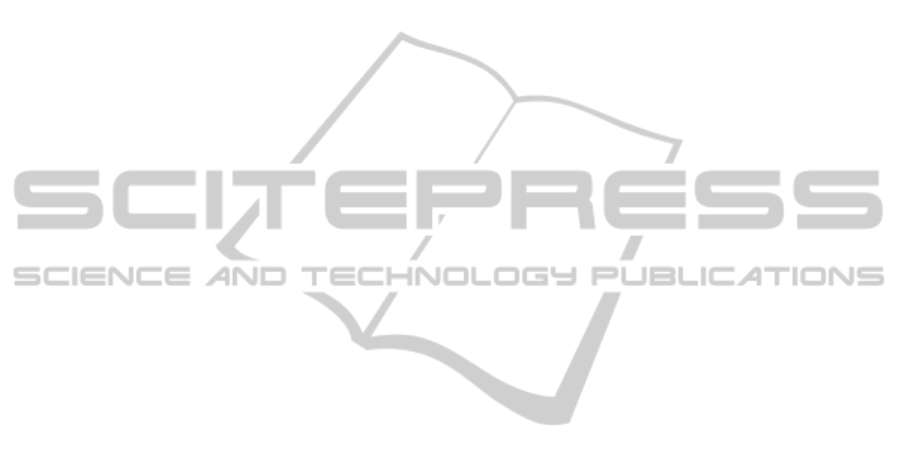
tabolically produced carbonic acid in the form of
carbon dioxide. Insects do not use a closed cir-
culatory system of arteries, veins and capillaries.
Instead of the lungs, they breathe through a seg-
mentally arranged system of spiracles and air-filled
tracheal tubes, which are ramified all over the body,
thus transporting aerial oxygen directly to tissue and
cells. The insect ‘‘blood’’ (haemolymph) circulates
between the three major body compartments (head,
thorax, abdomen), which are mutually inter-
connected and form an open body cavity, or
haemocoelic cavity (Jones, 1977); (Miller, 1997).
The dorsal vessel of adult insects consists of a
narrow elastic tube called the thoracic aorta and a
larger abdominal portion that is conventionally
called the insect heart in the strict sense. The
myocardium is segmentally prearranged, with
several pairs of usually incoming ostial valves,
perpendicular allatal muscles and pericardial
nutritive cells. In general, the insect heart is a tubular
organ, propagating waves of peristaltic contractions
in the forward direction (larvae), or alternatively in
both forward and backward direc-tions, which is
known as the heartbeat reversal. Recent
investigations show that, in comparison to the
human heart, the dorsal vessel of insects is a
relatively weak circulatory organ which is unable to
pump blood against any large barrier of mechanical
pressure (Sláma, 2000; 2012). Accordingly, the
insect heart is mostly used for mixing haemolymph
between the capital, thoracic and abdominal
compartments of the widely open body cavity. Due
to the limited, though very economic, pumping
ability of their heart, insects have evolved a number
of auxiliary circulatory adaptations such as the
accessory pulsatile organs of the appendages,
peristaltic pulsations of the intestine or strong extra-
cardiac pulsations in haemocoelic pressure (review
Sláma, 2008).
Insects and mammals are phylogenetically very
distant groups of animals (Prostomia and Deutero-
stomia) separated by millions of years of indepen-
dent evolutionary pathways. In spite of this, how-
ever, there exists well substantiated genetic evidence
that both insect (Drosophila) and human hearts share
some common morphogenetic principles (review by
Bodmer et al., (2005). Of particular interest in this
respect is a Tinman (Tin) gene containing a
transcriptional factor for the primordial heart in both
Drosophila and the human body (Ocorr et al.,
2007a); (Zeitouni et al., 2007). Comparisons
between the two phylogenetically distant circulatory
systems have been hampered for a long time by
superficially different anatomical and physiological
structures. Re-cently it has been found, however,
that both insect and human hearts are regulated by
similar, involun-tary and purely myogenic
mechanisms (Sláma and Lukáš, 2011). It also
appears that the rhythmicity of systolic cardiac
contractions in insects depends on a special
pacemaker nodus (Terminal regulatory no-dus)
(Sláma, 2006; 2012), which has a similar phy-
siological role to the atrioventricular, sinoatrial, or
Hiss bundle pacemaker nodi of the human heart
(Hampton, 2003). In addition to segmental, peristal-
tically propagated systolic contractions, certain
insect species have evolved a compact, conical
ventricle in the heart, characterised by a human-like
atrium and synchronic, not peristaltic, mode of
cardiac contractions (Sláma, 2010). Similarities be-
tween insect and human hearts prompted me to
investigate the possibility of similar responses with
respect to medicinal cardioactive drugs. The assays
were facilitated by the development of noninvasive,
touch-free, electrocardiographic methods for insects
(Sláma, 2003; 2006; 2012); (Sláma and Lukáš,
2011).
It appeared that the common cardioactive drugs
(noradrenaline, digitoxine, nitrates, Ca2+ ion
blockers, indeed produced heartbeat responses in
insects similar to those found in the human heart.
Encouraged by the results obtained in Drosophila
(Occor et al., 2007b); (Fink et al., 2009); (Sláma,
2010), I also looked at the hearts of some other flies.
Of particular interest was a family of hoverflies
(Syrphidae) including the presence of several real
champions in sustained flight. One species,
Episyrphus balteatus, revealed a quite uncommon,
anatomically and functionally human-like heart,
with a compact ventricle pumping the insect
‘‘blood’’ into an artery-like aorta (Sláma, 2013).
Here I describe new ECG data for an insect heart
and try to find possible analogies with the known
facts in human cardiology.
2 RESULTS AND DISCUSSION
2.1 Electrocardiography of Pupal
Hearts
The depolarization and repolarization electrical
potentials created by intensive contractions of the
compact human myocardium can be successfuly
recorded by external electrodes located at different
parts of the body. This represents the common prin-
ciple of ECG recordings in medicine (Hampton,
2003). The contracting insect myocardial cells exhi-
CARDIOTECHNIX2013-InternationalCongressonCardiovascularTechnologies
6