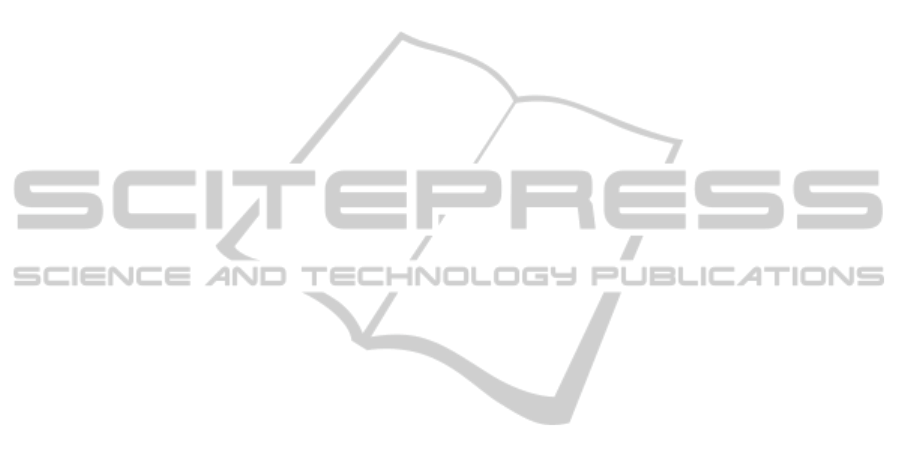
activation ratios between PC and SC (p>0.05).
4 DISCUSSION
Both MG and Sol demonstrated earlier onsets during
SC hopping. This represented a change from
potential feedback latency to a clear feedforward
response with onsets occurring within the defined
33 (+/- 7)ms window (Voigt et al., 1998).
Importantly, this was not matched by TibAnt.
Specifically, our findings demonstrate that in the
presence of a controlled environment and self-
regulation of the pending challenge and
consequences (i.e. the choice of hopping contact
time on a stable sleigh) individuals may choose a
feedforward strategy instead of the established co-
activation strategy. We observed a dynamic strategy
of pre-activation with an increased rate of activity of
the agonist muscle to develop force in time for
contact with the surface.
5 CONCLUSIONS
This study investigated the neural control of
consciously driven increase in joint stiffness during
submaximal hopping. We observed a stiffer hopping
performance driven by a feedforward strategy
confirming our hypothesis that internal challenges to
performance have their own unique motor strategies.
ACKNOWLEDGEMENTS
This research was partly supported by a grant from
the Neurotrauma Research Program – WA.
REFERENCES
Allison, G. T. 2003. Trunk Muscle Onset Detection
Technique For Emg Signals With Ecg Artefact.
Journal Of Electromyography And Kinesiology, 13,
209-216.
Allison, G. T., Marshall, R. N. & Singer, K. P. 1993. Emg
Signal Amplitude Normalization Technique In
Stretch-Shortening Cycle Movements. Journal Of
Electromyography And Kinesiology, 3, 236-244.
Blickhan, R. 1989. The Spring-Mass Model For Running
And Hopping. Journal Of Biomechanics, 22, 1217-
1227.
Bryant, A., Newton, R. & Steele, J. 2009. Successful
Feed-Forward Strategies Following Acl Injury And
Reconstruction. Journal Of Electromyography And
Kinesiology, 19, 988-997.
Dalleau, G., Belli, A., Viale, F., Lacour, J. & Bourdin, M.
2004. A Simple Method For Field Measurements Of
Leg Stiffness In Hopping. International Journal Of
Sports Medicine, 25, 170-176.
Farley, C. & Morgenroth, D. 1999. Leg Stiffness Primarily
Depends On Ankle Stiffness During Human Hopping.
Journal Of Biomechanics, 32, 267-273.
Ferris, D., Liang, K. & Farley, C. 1999. Runners Adjust
Leg Stiffness For Their First Step On A New Running
Surface. Journal Of Biomechanics, 32, 787-794.
Ferris, D. P. & Farley, C. T. 1997. Interaction Of Leg
Stiffness And Surface Stiffness During Human
Hopping. Journal Of Applied Physiology, 82, 15-22.
Fiolkowski, P., Bishop, M., Brunt, D. & Williams, B.
2005. Plantar Feedback Contributes To The
Regulation Of Leg Stiffness. Clinical Biomechanics,
20, 952-958.
Hobara, H., Inoue, K., Gomi, K., Sakamoto, M., Muraoka,
T., Iso, S. & Kanosue, K. 2010. Continuous Change In
Spring-Mass Characteristics During A 400 M Sprint. J
Sci Med Sport, 13, 256-61.
Hobara, H., Kanosue, K. & Suzuki, S. 2007. Changes In
Muscle Activity With Increase In Leg Stiffness During
Hopping. Neuroscience Letters, 418, 55-59.
Hodges, P., Van Den Hoorn, W., Dawson, A. &
Cholewicki, J. 2009. Changes In The Mechanical
Properties Of The Trunk In Low Back Pain May Be
Associated With Recurrence. Journal Of
Biomechanics, 42, 61-66.
Moritz, C., Greene, S. & Farley, C. 2004. Neuromuscular
Changes For Hopping On A Range Of Damped
Surfaces. J Appl Physiol, 96, 1996-2004.
Morris, S., Lay, B. & Allison, G. In Press: 2013.
Transversus Abdominis Is Part Of A Global Not Local
Muscle Synergy During Arm Movement. Human
Movement Science.
Moseley, G. L., Nicholas, M. K. & Hodges, P. W. 2004.
Does Anticipation Of Back Pain Predispose To Back
Trouble? Brain, 127, 2339-2347.
Müller, R. & Blickhan, R. 2010. Running On Uneven
Ground: Leg Adjustments To Altered Ground Level.
Human Movement Science, 29, 578-589.
Santello, M. 2005. Review Of Motor Control Mechanisms
Underlying Impact Absorption From Falls. Gait &
Posture, 21, 85-94.
Van Dieën, J. H., Selen, L. P. J. & Cholewicki, J. 2003.
Trunk Muscle Activation In Low-Back Pain Patients,
An Analysis Of The Literature. Journal Of
Electromyography And Kinesiology, 13, 333-351.
Voigt, Dyhre, P. & Simonsen 1998. Modulation Of Short
Latency Stretch Reflexes During Human Hopping.
Acta Physiologica Scandinavica, 163, 181-194.
Yeadon, M. R., King, M. A., Forrester, S. E., Caldwell, G.
E. & Pain, M. T. 2010. The Need For Muscle Co-
Contraction Prior To A Landing. J Biomech, 43, 364-
9.