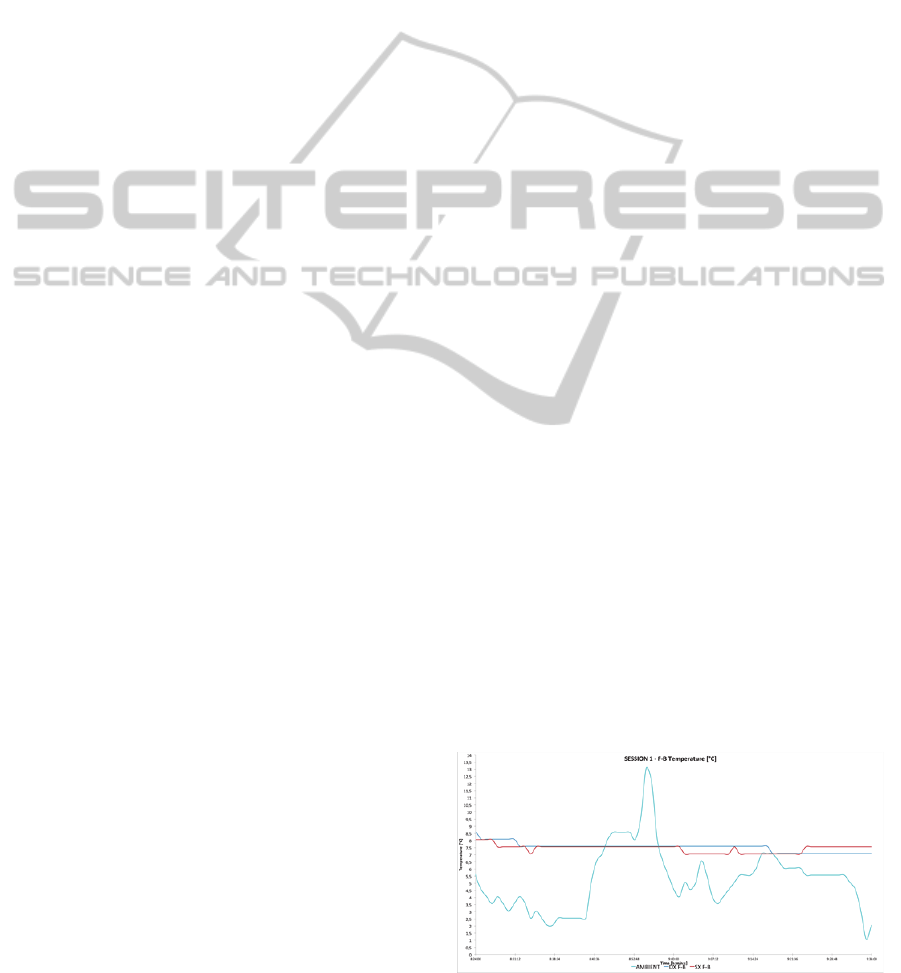
with respect to those for women; this denotes a
greater difficulty in ensuring a good level of thermal
comfort for the female gender. For both male and
female, further research can be carried out on
subjects with different tolerance to cold.
The application of this method to a larger
number of testers in a more standardised manner
(i.e. using the same skiing exercise pattern, the same
duration of each session and the same socks),
coupled with a statistical analysis, can assure great
improvements in products optimization, for a better
sport experience and a higher performance.
ACKNOWLEDGEMENTS
The authors would like to thank Calzaturificio Dal
Bello S.r.l. (Casella d'Asolo – TV – Italy) for the
support and funding of the research project.
REFERENCES
Afanasieva R. F. (1972) Hygienic basis for designing cold
protective clothing (Doctoral thesis), Moscow.
Bertaux E., Derler S., Zeng X., Koehl L., Ventenat V.
(2010) Textile, Physiological, and Sensorial
Parameters in Sock Comfort. Textile Research Journal
Vol 80(17): 1803–1810 DOI: 10.1177/004051
7510369409.
Enander A., Ljungberg A-S., Holmér I., (1979) Effects of
work in cold stores on man. Scand J Work Environ
Health 5, 195–204. Ergonomics 34, 687–720.
Fogarty A. L., Barlett R., Ventenar V., Havenith G.,
(2007). Regional foot sweat rates during a 65-minute
uphill walk with a backpack. The 12th International
Conference on Environmental Ergonomics, Mekjavic
I. B., Kounalakis S. N., Taylor N. A. S., (Eds.), 283–4,
Biomed d.o.o., Ljubljana, Piran.
Gran G (1957) Investigations on shoe climate and foot
comfort. J Soc Leather Techn & Chem 43, 182–97.
Goldman R., Kampmann B., (2007) Handbook on
clothing. Biomedical Effects of Military Clothing and
Equipment Systems. 2
nd
Edition.
Havenith G., Richards M. G., Wang X., Brode P., Candas
V., den Hartog E., Holmer I., Kuklane K., Meinander
H., Nocker W., (2008) Apparent latent heat of
evaporation from clothing: attenuation and “heat pipe”
effects. J Appl Physiol 104(1): 142-149.
Kuklane K., Holmér I., (1998) Effect of sweating on insu-
lation of footwear. Int J Occup Saf Ergon 4, 123–36.
Kuklane K., Holmér I., Giesbrecht G., (1999) Change of
footwear insulation at various sweating rates. Appl
Human Sci 18, 161–8.
Kuklane K., Holmér I., Giesbrecht G., (2000) One week
sweating simulation test with a thermal foot model. In:
The Third International Meeting on Thermal Manikin
Testing, Nilsson H and Holmér I (Eds.), 106–13,
National Institute for Working Life, Stockholm.
Kuklane K., (2009) Protection of Feet in Cold Exposure.
Luczak H., (1991). Work under extreme conditions.
Oakley E. H. N., (1984) The design and function of mili-
tary footwear: a review following experiences in the
South Atlantic. Ergonomics 27, 631–7.
Pezzoli A., Baldacci A., Cama A., Faina M., Dalla Vedova
D., Besi M., Vercelli G., Boscolo A., Moncalero M.,
Cristofori E., Dalessandro M., (in press) Wind-wave
interactions in enclosed basins: the impact on the sport
of rowing. In: Physics of Sport. Ed. Ecole
Polytechnique de Paris. Paris.
Pezzoli A., Cristofori E., Gozzini B., Marchisio M.,
Padoan J., (2012) Analysis of the thermal comfort in
cycling athletes. Procedia Engineering, 34:433-438.
Pezzoli A., Moncalero M, Boscolo A, Cristofori E,
Giacometto F, Gastaldi S, Vercelli G (2010) The
meteo-hydrological analysis and the sport
performance: which are the connections? The case of
the XXI Winter Olympic Games, Vancouver 2010.
Journal of Sports Medicine and Physical Fitness,
50:19-20.
Rintamaaki H., Hassi J., (1989) Thermal physiology and
cold protection of feet with two types of rubber boots.
Arctic Rubber, Scandinavian Rubber Conference,
Tampere.
Taylor N. A. S., Galdwell J. N., Mekjvic I. B., (2006).The
sweating foot: local differences in sweat secretion
during exercise-induced hyperthermia. A viat Space
Environ Med 77, 1020–7.
The Thermal Environment Laboratory, Division of
Ergonomics and Aerosol Technology, Department of
Design Sciences, Faculty of Engineering, Lund
University, Box 118, SE-221 00 Lund, Sweden.
Wang F., del Ferraro S., Lin L. Y., Sotto Mayor T.,
Molinaro V., Ribeiro M., Gao C., Kuklane K., Holmer
I., (2012). Localised boundary air layer and clothing
evaporative resistances for individual body segments.
Ergonomics 55(7):799-812. doi: 10.1080/00140139.
2012.668948.
APPENDIX
Graph 1: Session 1, F-B TEMPERATURE [°C].
icSPORTS2013-InternationalCongressonSportsScienceResearchandTechnologySupport
178