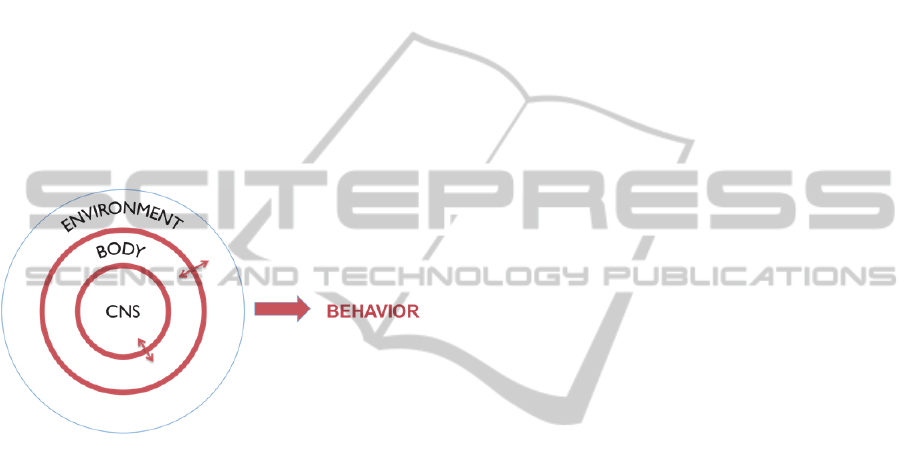
elements (Figure 2). This hypothesis has two logic
consequences. The first is that interaction is a crucial
part of the system. The second is that the whole
system is much more that the sum of its parts.
As applied to Neurorobotics, these concepts have
important practical implications. The first one is that
neurorobots should be real structures that interact
with real environment. Use of simulation, in this
context, should be limited only to the first stages of
robot design. In fact, the interaction between real
structures (e.g. contact with the ground during
walking) embeds physical phenomena that are yet to
be accurately represented in simulation. Another
important implication is that even very complex
behaviours can be potentially studied using a few
simple elements in interactions to each other
(Giszter, 2001).
Figure 2: Holistic view of the biological motor control
system and the fundamental role of the interactions in the
generation of behaviours.
How can the principles of Neurorobotics be used
to develop more effective rehabilitation and
neurorehabilitation machines? Let’s consider the
case of rehabilitation of locomotion.
As opposed to a classical rehabilitation
engineering approach, which aims at solving the
problem in functional terms (e.g. by developing a
neuroprosthesis that allows restoring gait), the
neurorobotics approach is strongly based on
preliminary observation of the mechanisms that
emerge in a neurorobot. These mechansims are the
result of the interaction between the three main
elements resembling those of humans, i.e. the
control system (brain), the plant (body) and the
environment (Figure 3). The key point is that some
of these interactions may have not been modelled
previously, but emerge naturally from the correct
implementation of neural control into the
biomechanical structures. The effects of them can be
studied in deep detail at different levels, because
robotic structures offer many advantages for
experimental observation with respect to human
subjects.
Practically, this process includes two main
actions. The first is to create a neurorobot that
embeds the main known physiological principles of
human locomotion. The second is to extract, from
the analysis of the behaviour of the robot, clues that
can be turned into design principles for rehabilitation
machines.
As for the first action, i.e. the development of the
neurorobot, the following main steps should be
followed:
1. The basic biomechanical and neural principles of
human locomotion are first translated to a
human-like neurorobot, represented by a
humanoid (or part of it).
2. The functionality of walking is then tested and
mechanisms refined in an iterative fashion, in
order to obtain intelligent behaviour, i.e. human-
like walking.
3. Once stable and human-like walking is achieved,
the different levels of interaction of the
neurorobot (brain-body interaction, body-
environment interaction) are analysed.
4. These interaction mechanisms are then
formalized in order to understand the cause-
effect relation between internal control and
functional behaviour.
As for the second action, i.e. transferring the
acquired knowledge to the rehabilitation scenario,
different approaches can be envisioned. The
neurorobot can be include either mechanisms of a
healthy subject, or can be modified to match a
specific known motor disability.
In the “healthy neurorobot” scenario, once the
neurorobot is developed, the principles of actuation
implemented in the machine are prone to be
transferred to rehabilitation machines. For instance,
feed-forward control strategies implemented in the
robot can be used to implement biologically based
neuro-prosthetic control algorithms. In a similar
fashion, local reflex-based robotic principles, which
describe the reaction of the robot joint to the
interaction with the environment, may be translated
into control algorithms for lower limb prostheses.
In the “pathologic robot” scenario the efforts are
devoted at reproducing a specific impaired
behaviour, by modifying internal control or
biomechanical parameters of the robot. In this case,
different rehabilitation potentialities can be
identified. If the pathologic behaviour is successfully
reproduced, the cause-effects relation between the
affected biological principle and the functional
HumanoidsMeetRehabilitation-ConceptandPotential
215