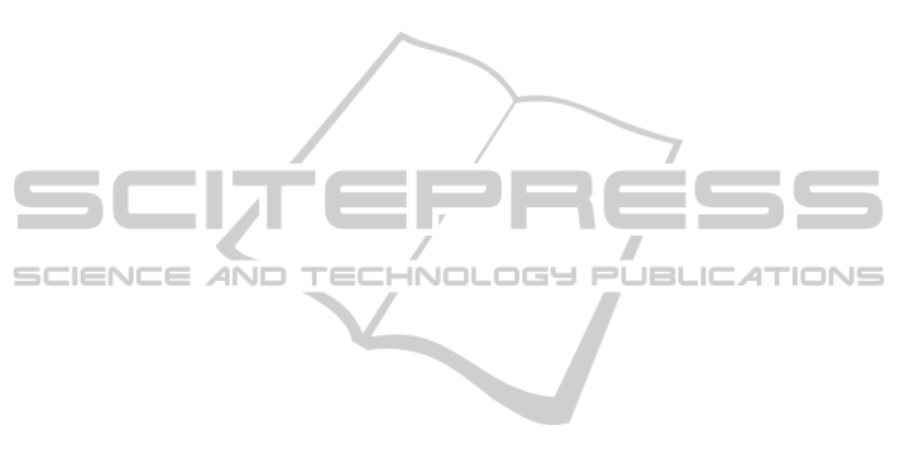
4.2 Results
As shown in Figure 6, the signal obtained with our
off-the-person approach is clearly a lead I derivation.
The average cosine distance of nearly zero between
the off-the-person waveform and the lead I waveform
of the medical-grade device, together with the low
standard deviation across the overall set of subjects,
allow us to conclude that both signals are fully corre-
lated. This is further reinforced by the visual observa-
tion of the data; in Figure 7 we depict an overlay with
the segmented individual heartbeat waveforms for one
of the tested subjects. The off-the-person data is rep-
resented in blue, while the on-the-person data for each
of the leads is represented in green; in this case, the
off-the-person lead and the lead I data present an al-
most exact match.
Analyzing the case-by-case statistics, we are able
to observe that for some of the subjects, leads II, aVF,
aVR, and V3-V6 also exhibit a low average distance
to the waveform obtained using the off-the-person ap-
proach, and hence a high morphological similarity.
For example in Figure 7, V3 and V4 are quite simi-
lar to the off-the-person lead. The distributions of the
individual distances between our off-the-person lead
and each of the medical-grade leads for the overall
population can be found in Figure 8.
5 DISCUSSION AND FUTURE
WORK
Electrocardiography (ECG) has progressed a long
way since it was first introduced in the clinical prac-
tice. In the recent years, an increasingly growing
community has focused on improving the usability of
ECG equipment, and while most of the work has been
targeting wearable form factors (e.g. t-shirts), our
work has been pivoting towards what can be classified
as an off-the-person approach. In this paper we have
proposed a taxonomy of ECG data acquisition meth-
ods with respect to their intrusiveness level, described
an off-the-person sensor designed for ECG data ac-
quisition at the hands and fingers using dry electrodes,
and provided experimental results regarding the com-
parison between the off-the-person approach and con-
ventional medical-grade equipment.
Comparative tests have shown that the signals
obtained through our off-the-person approach are
matched to the conventional lead I derivation, and
that even without skin preparation or the use of con-
ductive paste to lower the impedance with the skin,
the morphology of the heartbeat waveform can be re-
trieved. Our work is targeted at ECG data acquisition
in a pervasive framework, by providing a simplified
sensor setup that can be used for everyday monitor-
ing. The applicability of our pervasive ECG approach
is not bound to the healthcare and clinical domains,
given that the ECG and derived measurements are
also appealing in a wide range of emerging applica-
tions, which include self-management, affective com-
puting (Medina, 2009) or even security (Lourenc¸o
et al., 2011)(Silva et al., 2013).
Future work will focus on further validating our
approach by increasing the number of tested sub-
jects, and also in the evaluation of contactless off-the-
person approaches targeting the evaluation of the rela-
tion between the signals obtained using such methods,
and the signals obtained using conventional methods.
ACKNOWLEDGEMENTS
This work was partially funded by Fundac¸
˜
ao
para a Ci
ˆ
encia e Tecnologia (FCT) under grants
PTDC/EEI-SII/2312/2012, SFRH/BD/65248/2009
and SFRH/PROTEC/49512/ 2009, whose support the
authors gratefully acknowledge. We would also like
to thank to Hospital de Santa Marta, Dr. Rui Cruz
Ferreira, Rui C
´
esar das Neves, and Jos
´
e Guerreiro for
their technical support during the development of this
work.
REFERENCES
Barold, S. (2003). Willem Einthoven and the birth of clin-
ical electrocardiography a hundred years ago. Card.
Electrophysiol. Rev., 7:99–104.
Besterman, E. and Creese, R. (1979). Waller - pioneer of
electrocardiography. British Heart Journal, 42:61–64.
Canento, F., Lourenc¸o, A., Silva, H., Fred, A., and Raposo,
N. (2013). On real time ECG algorithms for biometric
applications. In Proc. of the 6th BIOSIGNALS Conf.
Chi, Y., Jung, T.-P., and Cauwenberghs, G. (2010).
Dry-contact and noncontact biopotential electrodes:
Methodological review. IEEE Rev. Biomed. Eng.,
3:106 –119.
Chung, E. K. (2000). Pocketguide to ECG Diagnosis.
Blackwell Publishing.
de Isla, L. P., Lennie, V., Quezada, M., Guinea, J., Arce,
C., Abad, P., Saltijeral, A., Carolina, N., Crespo, J.,
Gonz
´
alvez, B., Macia, A., and Zamorano, J. (2011).
New generation dynamic, wireless and remote car-
diac monitorization platform. Int’l Journ. of Card.,
153(1):83–85.
Drew, B. J., Califf, R. M., Funk, M., Kaufman, E. S., Kru-
coff, M. W., Laks, M. M., Macfarlane, P. W., Sommar-
gren, C., Swiryn, S., and Van Hare, G. F. (2004). Prac-
Off-the-PersonElectrocardiography
105