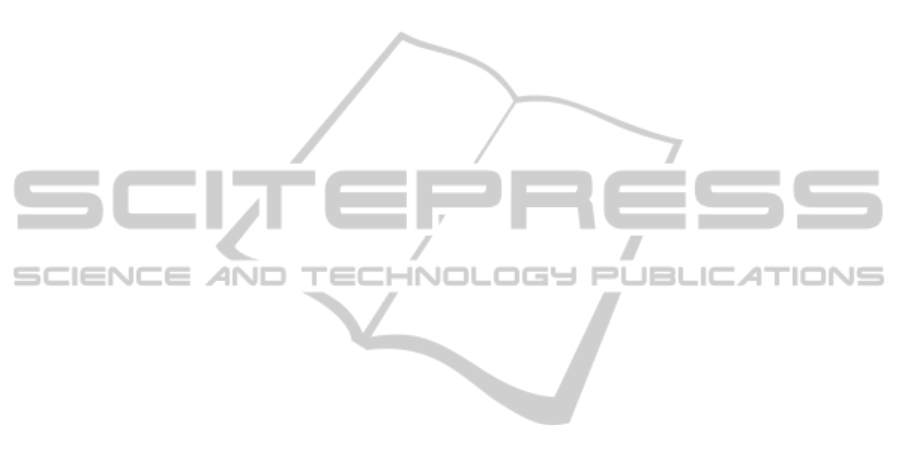
Iosa, M., Tamburella, F., Moreno, J. C., Collantes, I., As
´
ın,
G., Aloise, F., Pisotta, I., Muzzioli, L., Mattia, D.,
Molinari, M., Pons, J. L., and Cincotti, F. (2012). Neu-
rorehabilitation after stroke: a new tool for a top-down
approach. In Terzo Congresso Gruppo Nazionale
Bioingegneria (GNB 2012).
Kao, P.-C. and Ferris, D. P. (2009). Motor adaptation during
dorsiflexion-assisted walking with a powered orthosis.
Gait & posture, 29(2):230–236.
Kao, P.-C., Lewis, C. L., and Ferris, D. P. (2010a). Invariant
ankle moment patterns when walking with and with-
out a robotic ankle exoskeleton. Journal of biome-
chanics, 43(2):203–209.
Kao, P.-C., Lewis, C. L., and Ferris, D. P. (2010b). Short-
term locomotor adaptation to a robotic ankle ex-
oskeleton does not alter soleus hoffmann reflex ampli-
tude. Journal of neuroengineering and rehabilitation,
7(1):33.
Krebs, H. I. and Hogan, N. (2006). Therapeutic robotics:
A technology push. Proceedings of the IEEE,
94(9):1727–1738.
Kwa, H. K., Noorden, J. H., Missel, M., Craig, T., Pratt,
J. E., and Neuhaus, P. D. (2009). Development of the
ihmc mobility assist exoskeleton. In Robotics and Au-
tomation, 2009. ICRA’09. IEEE International Confer-
ence on, pages 2556–2562. IEEE.
Moreno, J. C., Brunetti, F., Rocon, E., and Pons, J. L.
(2008). Immediate effects of a controllable knee an-
kle foot orthosis for functional compensation of gait
in patients with proximal leg weakness. Medical &
biological engineering & computing, 46(1):43–53.
Perry, J. (1992). Phases of gait. In Gait Analysis: Normal
and Pathological Function, pages 9–16. Slack, Tho-
rofare, NJ.
Saito, Y., Kikuchi, K., Negoto, H., Oshima, T., and
Haneyoshi, T. (2005). Development of externally
powered lower limb orthosis with bilateral-servo ac-
tuator. In Rehabilitation Robotics, 2005. ICORR
2005. 9th International Conference on, pages 394–
399. IEEE.
Sawicki, G. S. and Ferris, D. P. (2008). Mechanics and ener-
getics of level walking with powered ankle exoskele-
tons. Journal of Experimental Biology, 211(9):1402–
1413.
Sawicki, G. S. and Ferris, D. P. (2009). A pneumatically
powered knee-ankle-foot orthosis (kafo) with myo-
electric activation and inhibition. Journal of neuro-
engineering and rehabilitation, 6(1):23.
Stauffer, Y., Allemand, Y., Bouri, M., Fournier, J., Clavel,
R., Metrailler, P., Brodard, R., and Reynard, F. (2009).
The walktrainer-a new generation of walking reeduca-
tion device combining orthoses and muscle stimula-
tion. Neural Systems and Rehabilitation Engineering,
IEEE Transactions on, 17(1):38–45.
Van der Kooij, H., Veneman, J., and Ekkelenkamp, R.
(2006). Compliant actuation of exoskeletons. Mo-
bile robotics-towards new applications, Mammendorf.
ISBN, pages 978–3.
Ward, J. A., Hitt, J., Sugar, T., and Bharadwaj, K. (2006).
Dynamic pace controller for the robotic gait trainer.
ASME.
Wheeler, J. W., Krebs, H. I., and Hogan, N. (2004). An
ankle robot for a modular gait rehabilitation system.
In Intelligent Robots and Systems, 2004.(IROS 2004).
Proceedings. 2004 IEEE/RSJ International Confer-
ence on, volume 2, pages 1680–1684. IEEE.
NEUROTECHNIX2013-InternationalCongressonNeurotechnology,ElectronicsandInformatics
166