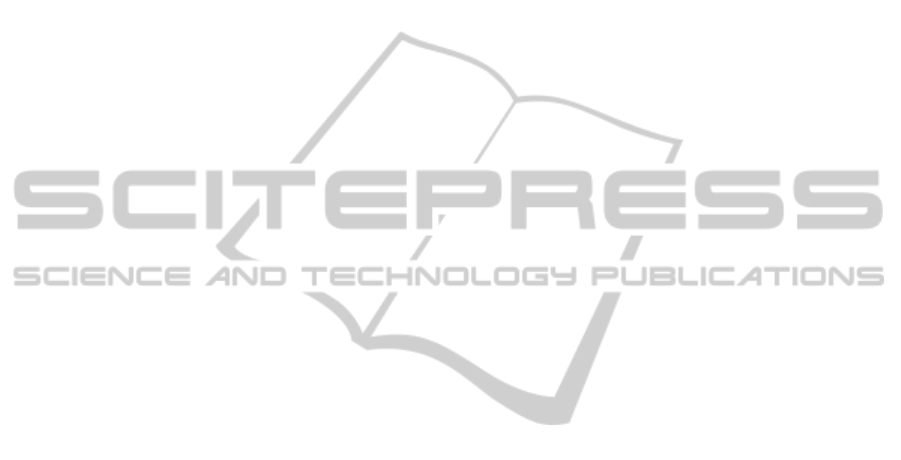
Montagner, A., Frisoli, A., Borelli, L., Procopio, C.,
Bergamasco, M., Carboncini, M. C. & Rossi, B., Year.
A pilot clinical study on robotic assisted rehabilitation
in VR with an arm exoskeleton deviceed.^eds. Virtual
Rehabilitation, 2007, 57-64.
Morris, S. L., Dodd, K. J. & Morris, M. E., 2004.
Outcomes of progressive resistance strength training
following stroke: a systematic review. Clin Rehabil,
18, 27-39.
Norouzi-Gheidari, N., Archambault, P. S. & Fung, J.,
2012. Effects of robot-assisted therapy on stroke
rehabilitation in upper limbs: systematic review and
meta-analysis of the literature. Journal of Rehabilitation
Research and Development, 49, 479-96.
Patton, J. L., Kovic, M. & Mussa-Ivaldi, F. A., 2006a.
Custom-designed haptic training for restoring reaching
ability to individuals with poststroke hemiparesis. J
Rehabil Res Dev, 43, 643-56.
Patton, J. L. & Mussa-Ivaldi, F. A., 2004. Robot-assisted
adaptive training: custom force fields for teaching
movement patterns. IEEE Trans Biomed Eng, 51, 636-46.
Patton, J. L., Stoykov, M. E., Kovic, M. & Mussa-Ivaldi,
F. A., 2006b. Evaluation of robotic training forces that
either enhance or reduce error in chronic hemiparetic
stroke survivors. Experimental Brain Research, 168,
368-383.
Prange, G. B., Jannink, M. J. A., Groothuis-Oudshoorn, C.
G. M., Hermens, H.J. & Ijzerman, M. J., 2006.
Systematic review of the effect of robot-aided therapy
on recovery of the hemiparetic arm after stroke.
Journal of Rehabilitation Research and Development,
43, 171-183.
Reinkensmeyer, D. J., 2009. Robotic Assistance for Upper
Extremity Training after Stroke. In A. Gaggioli, E. A.
Keshner, P. L. Weiss & G. Riva (eds.) Advanced
Technologies in Rehabilitation: Empowering
Cognitive, Physical, Social and Communicative Skills
through Virtual Reality, Robots, Wearable Systems
and Brain-Computer Interfaces. 25-39.
Reinkensmeyer, D. J., Emken, J. L. & Cramer, S. C.,
2004. Robotics, motor learning, and neurologic
recovery. Annual Review of Biomedical Engineering,
6, 497-525.
Rozario, S. V., Housman, S., Kovic, M., Kenyon, R. V. &
Patton, J. L., 2009. Therapist-mediated post-stroke
rehabilitation using haptic/graphic error augmentation.
Conf Proc IEEE Eng Med Biol Soc, 2009, 1151-6.
Scott, S. H. & Dukelow, S. P., 2011. Potential of robots as
next-generation technology for clinical assessment of
neurological disorders and upper-limb therapy.
Journal of Rehabilitation Research and Development,
48, 335-353.
Shirzad, N., Van Der Loos, H. F. M. & Ieee, 2012. Error
Amplification to Promote Motor Learning and
Motivation in Therapy Robotics. 2012 Annual
International Conference of the Ieee Engineering in
Medicine and Biology Society. 3907-3910.
Sivan, M., O'connor, R. J., Makower, S., Levesley, M. &
Bhakta, B., 2011. Systematic review of outcome
measures used in the evaluation of robot-assisted
upper limb exercise in stroke. J Rehabil Med, 43, 181-
9.
Squeri, V., Vergaro, E., Brichetto, G., Casadio, M.,
Morasso, P. G., Solaro, C. & Sanguineti, V., Year.
Adaptive robot training in the rehabilitation of
incoordination in Multiple Sclerosis: A pilot
studyed.^eds., Noordwijk, 364-370.
Squeri, V., Vergaro, E., Brichetto, G., Casadio, M.,
Morasso, P. G., Solaro, C., Sanguineti, V. & Ieee,
2007b. Adaptive robot training in the rehabilitation of
incoordination in Multiple Sclerosis: a pilot study.
2007 Ieee 10th International Conference on
Rehabilitation Robotics, Vols 1 and 2. 364-370.
Teulings, H. L., Contrerasvidal, J. L., Stelmach, G. E. &
Adler, C. H., 1997. Parkinsonism reduces coordination
of fingers, wrist, and arm in fine motor control.
Experimental Neurology, 146, 159-170.
Vergaro, E., Squeri, V., Brichetto, G., Casadio, M.,
Morasso, P., Solaro, C. & Sanguineti, V., 2010.
Adaptive robot training for the treatment of
incoordination in Multiple Sclerosis. J Neuroeng
Rehabil, 7, 37.
Wang, F., Barkana, D. E. & Sarkar, N., 2010. Impact of
visual error augmentation when integrated with assist-
as-needed training method in robot-assisted
rehabilitation. IEEE Trans Neural Syst Rehabil Eng,
18, 571-9.
Wei, Y., Bajaj, P., Scheidt, R., Patton, J. & Ieee, 2005.
Visual error augmentation for enhancing motor
learning and rehabilitative relearning. 2005 Ieee 9th
International Conference on Rehabilitation Robotics.
New York: Ieee, 505-510.
Weightman, A., Preston, N., Levesley, M., Holt, R., Mon-
Williams, M., Clarke, M., Cozens, A. J. & Bhakta, B.,
2011. Home based computer-assisted upper limb
exercise for young children with cerebral palsy: a
feasibility study investigating impact on motor control
and functional outcome. Journal of Rehabilitation
Medicine, 43, 359-363.
Wolpert, D. M., Ghahramani, Z. & Jordan, M. I., 1995. An
internal model for sensorimotor integration. Science,
269, 1880-1882.
Wu, C. Y., Trombly, C. A., Lin, K. C. & Tickle-Degnen,
L., 2000. A kinematic study of contextual effects on
reaching performance in persons with and without
stroke: Influences of object availability. Archives of
Physical Medicine and Rehabilitation, 81, 95-101.
NEUROTECHNIX2013-InternationalCongressonNeurotechnology,ElectronicsandInformatics
174