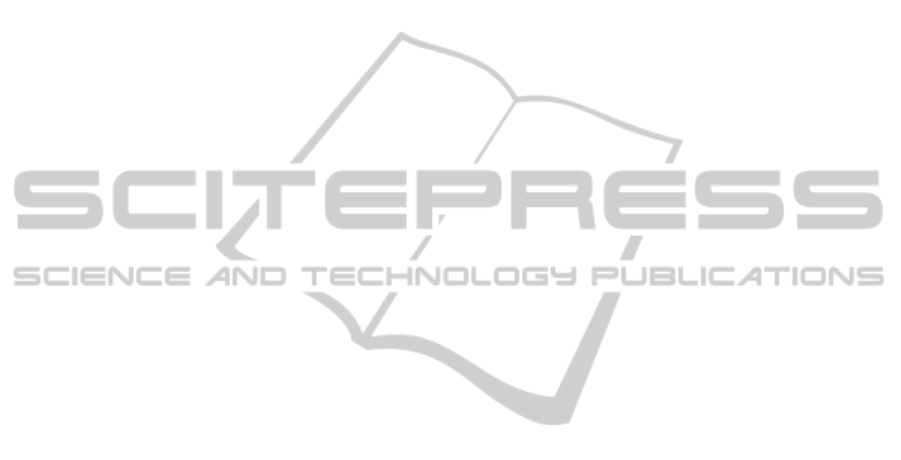
2.1 Questionnaires
A web-based questionnaire containing questions on
all ICF domains, was composed to evaluate overall
arm function and problems people encounter in daily
life. This questionnaire was distributed among
various groups of people with neuromuscular
diseases, namely Duchenne muscular dystrophy
(DMD), fascioscapulohumeral dystrophy (FSHD),
limb-girdle muscular dystrophy (LGMD) and spinal
muscular atrophy (SMA).
2.2 3D Kinematics and EMG
Motion analysis and electromyography (EMG) data
from various tasks (e.g. shoulder abduction/flexion,
reaching and hand to mouth movement) were
recorded during unsupported movement and during
supported movement with a passive Sling arm
support (Focal Meditech). In both cases the subject
was asked to move the dominant hand from an initial
position resting on a table in the sagittal plane to a
target placed at a distance of a stretched arm, at
shoulder height and one shoulder width on the
ipsilateral side. The movements were recorded with
a 3D camera Motion Capture system (Vicon).
Reflective markers were attached on the subject’s
body following the guidelines of the Vicon Upper
Limb model. These data were subsequently used in
simulations with a multi-body model of the arm to
calculates joint moments. EMG data were obtained
from biceps brachii, deltoid, triceps brachii,
trapezius, pectoralis and latissimus dorsi muscles
and were normalized as percentage of the EMG
during maximum voluntary contraction.
2.3 Muscle-skeletal Simulations
The coordinates of the reflective markers during the
unsupported and supported ipsilateral reaching
movements were used to drive the simulation model
in the AnyBody Modeling System (AnyBody
Technology). With the subject’s anthropometric
information derived from marker coordinates, the
software’s GaitFullBody model was scaled
according to body length and mass among others.
An inverse dynamic analysis was then carried out to
calculate the net joint moments at the shoulder and
elbow. The analysis on the unsupported movement
consisted of two parts: a normal gravity situation
and a simulated zero gravity situation, in which the
same motion data for the unsupported movement
were used but gravity was set to zero in AnyBody’s
model parameters. As a result the outputs of the
calculation were the net joint moments in three
conditions: I control, II gravity compensation with
Sling and III zero gravity environment. These
conditions were chosen to assess the influence of
gravity compensation (I vs. II), the influence of a
zero gravity environment (I vs. III) and the
difference between gravity compensation induced
either by a mechanism or resulting from a zero
gravity environment (II vs. III)(Essers, 2013).
2.4 Ambulatory Performance
To evaluate the effect of arm supporting devices in a
daily life setting, a protocol for monitoring the arm
activity outside a laboratory setting was developed.
A tri-axial accelerometer (MOX, Maastricht
Instruments) was placed on the upper arm just above
the elbow. The acceleration signals were post-
processed to obtain elevation and intensity of upper
arm movements. These data give an indication on
how and how often the arm support is used in daily
life (Annegarn, 2012).
3 RESULTS
Preliminary results for the various studies are
shown.
3.1 Questionnaires
In total of 315 boys/men with DMD, 88 with FSHD,
61 with LGMD and 73 with SMA participated.
Preliminary data show that pain, stiffness and
functional limitations increased with age in DMD.
Data of FSHD, LGMD and SMA are being
analysed.
3.2 3D Kinematics and EMG
The maximum shoulder elevation angles and the
minimal and maximal elbow flexion angles were
analysed in a group of 11 people with FSHD and in
a group of 8 healthy controls. The data depicted in
figure 1 represent the shoulder elevation angles of
the healthy control group and the data of the FSHD
subjects. Significant differences between the
shoulder angles of the FSHD and the healthy control
group were found for the shoulder elevation angle
during the abduction and flexion tasks and during
the two reaching tasks. Of the 11 subjects, only two
were able to elevate the arm above 90 degrees. The
EMG data showed higher percentages in the FSHD
FSHD group compared to the control group.