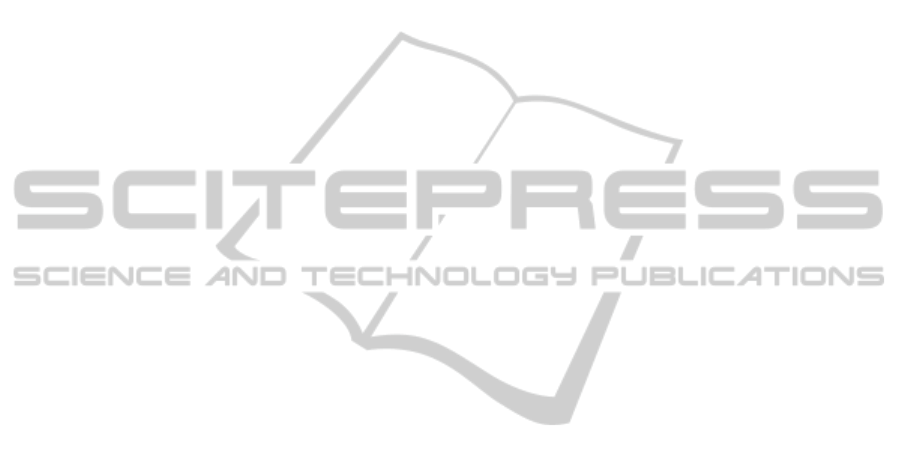
optimized and the performance of this coating on
bulk TAS sample is demonstrated successfully.
Proper design of a copper fiber holder for
cryogenic temperature application is investigated
and realized. Cryogenic test with gold coated TAS
fibers mounted in this holder has proven that the
TAS fibers successfully survives cool down to 40
K without visible degradation or failure. Five
short wavelength TAS single mode waveguides
with gold absorption coating but without AR
coating are integrated in the fiber holder and
characterised by cross core scan and FFI.
ACKNOWLEDGEMENTS
This project was funded by the European space
Agency under the contract No. 20914/07/NL/CP.
The authors would like to thank the
Optoelectronics Research Centre of University of
Southampton for the modelling of the cladding
mode suppression and Netherlands Institute for
Space research (SRON) for providing their
Cryogenic facility.
REFERENCES
Woolf, N., and Angel, J., 1998 “Astronomical searches
for earth-like planets and signs of life,”
Astron.Astrophys.36, pp. 507-537.
Kaltenegger, L., Fridlund, M., 2005, “The Darwin
mission: Search for extra-solar planets”, Advances
in Space Research 36, pp 1114-1122.
Bracewell, R. N., 1978 “Detecting nonsolar planets by
spinning infra-red interferometer”, Nature 274, pp
780-781.
Angel, J. R. P., Cheng, A. Y. S., and Woolf, N. J., 1978
“A space telescope for infra-red spectroscopy of
earth like planets”, Nature 322, pp 341-434.
Cheng, L. K., Faber, A. J., Gielesen, W., Boussard-
Pledel, C., Houizot, P., Lucas, J., and Pereira Do
Carmo, J., 2005 “Test results of the infrared single-
mode fiber for the DARWIN mission,” Proceedings
of SPIE 5905, 59051F.
Cheng, L. K., Faber, A. J., Gielesen, W., Lucas, J.,
Boussard-Plédel, C., Houizot,P., and Pereira do
Carmo, J., 2006 “Development of broadband
infrared single-mode fibers for the DARWIN
mission,” Proceedings of SPIE 6268, 62682F.
Faber, A. J., Cheng, L. K., Gielesen, W. L. M.,
Boussard-Plédel, C., Houizot, P., Danto,S., Lucas,
J., and Pereira Do Carmo, J., 2006, “Single Mode
Chalcogenide Glass Fiber as Wavefront Filter for
the Darwin Planet Finding Mission,” Proceedings
of ‘6th International. Conference. on Space Optics’
ESTEC, pp. 27-30.
Cheng, L. K. et al., 2009, “Development of infrared
single-mode fibers for 2 wavelength bands of the
Darwin mission: Test results of prototypes”,
Proceedings of SPIE 7420.
Houizot, P., Boussard-Plédel, C., Faber, A. J., Cheng,
L. K., Bureau, B., Van Nijnatten, P. A., Gielesen,
W. L. M., Pereira do Carmo, J., and Lucas, J., 2007,
“Infrared single mode chalcogenide glass fiber for
space, Optics Express 15 (19), pp 12529-12538.
Zhukova, L., Korsakov, A., Chazov, A., Vrublevsky, D.
and Zhukov, V., 2012, “Photonic crystalline IR
fibers for the spectral range of 2–40 μm”, Applied
Optics 51, pp. 2414-2418.
Spronck, J. F. P., Fischer, D. A. and Kaplan, Z A.,
2012, "Use and limitations of single-and multi-
mode optical fibers for exoplanet detection."
Optical Fibers/Book 3.
PHOTOPTICS2014-InternationalConferenceonPhotonics,OpticsandLaserTechnology
20